NF-κB pathway in colitis-associated cancers
Introduction
Colorectal cancer (CRC) is one of the most commonly diagnosed cancers. In developed countries, this multifactorial disease is the third most commonly diagnosed cancer in males, the second in females, and the second and third leading cause of cancer death in males and females, respectively (1).
Factors that may increase the risk of colon cancer include advanced age, certain genetic features, (positive) smoking status, diet, the extent of physical activity, virus infection, environmental influences, intake of exogenous hormones, alcohol ingestion, and inflammation (2,3). Over the past decade, several notable studies have revealed a connection between inflammation and colon carcinogenesis (4,5). Both inflammatory bowel disease (IBD)-associated and sporadic CRC are characterized by a dysplastic cancer sequence; multiple mutations are required before carcinoma develops.
Epidemiological studies have shown that chronic inflammation predisposes patients to cancers of many organs, and administration of non-steroidal anti-inflammatory drugs (NSAIDs) decreases the incidence of CRC (6,7). Although many reports have documented the critical link between inflammation and development of colon cancer, our knowledge of the underlying mechanisms remains incomplete. Of the various signaling pathways involved in colonic inflammation, the NF-κB pathway is central. NF-κB proteins are involved in the control of many normal cellular and physiological processes, including immune and inflammatory responses, developmental processes, cellular growth, and apoptosis (8-10). A relationship between cancer development and NF-κB signaling is becoming clear (11), and the present review will focus on the role played by this signaling pathway in colorectal carcinogenesis. Also, NF-κB-targeting therapies that may aid in treatment of CRC will be discussed.
The NF-B pathway
Overview
Nuclear Factor-Kappa B (NF-κB) proteins are transcription factors that regulate many physiological processes, including the innate and adaptive immune responses, apoptosis, cell proliferation, inflammation, and malignant transformation. Deregulation of normal NF-κB activity, such as expression of an abnormal form of the protein, or interference with transcriptional activity from the normal gene, has been shown to be involved in development of leukemia, lymphomas, and solid tumors (12). NF-κB family members also influence tumor development via activation of apoptosis-resistant genes; this creates resistance to radiotherapy and chemotherapy (13).
Structure, role and activation
Members of NF-κB are transcriptional factor and can either induce or repress gene expression by binding to specific DNA sequences in promoters of target genes; these sequences are termed κB elements. Mammalian cells express five members of the NF-κB family: RelA (p65), RelB, c-Rel, p50/p105 (NF-κB1), and p52/p100 (NF-κB2). Various NF-κB complexes containing homo- or hetero-dimers of these proteins can be formed. In most cell types, NF-κB complexes are retained in the cytoplasm by the actions of a family of inhibitory proteins termed inhibitors of NF-κB (IκB) (14) (Figure 1). The activation of the NF-κB is based on the induction of the IκB by IκB kinase (IKK). IKK is a protein complex composed of catalytic subunits, IKKα, IKKβ and a regulatory subunit named NF-κB essential modulator (NEMO) or IKKγ.
The transcriptional activity of NF-κB is regulated by two pathways, termed the canonical and non-canonical pathway. The canonical pathway requires activation by the RelA/p50 dimer and such activation is lacking if the relevant dimers are sequestered in the cytoplasm by inhibitory IκB proteins (14,15). Upon stimulation of various types, including that mediated by cytokines, growth factors, viral or bacterial proteins, or oxidative stress, IκB proteins are phosphorylated on specific serine residues by IKKα/IKKβ complex leading to ubiquitin-dependent IκB degradation by the 26S proteasome (16). The RelA/p50 dimers are thus free to translocate to the nucleus and to bind therein to the κB elements, found in promoters and enhancers of target genes (Figure 1). The other pathway, the alternative or non-canonical pathway, is triggered by certain members of the TNF cytokine family and results in selective activation of RelB/p52 dimers. The non-canonical pathway causes activation of IKKα by the NF-κB-inducing kinase (NIK), followed by phosphorylation of the NF-κB p100 subunit by IKKα. This, in turn, results in proteasome-dependent processing of p100 to p52, triggering activation of RelB/p52 heterodimers that target distinct κB elements (14).
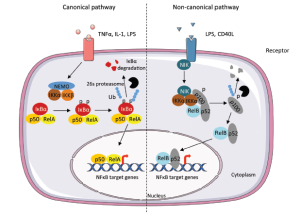
NF-B in colon carcinogenesis
Colitis-associated cancer
Colitis-associated cancer (CAC) is the subtype of CRC that is associated with IBDs including ulcerative colitis (UC) and Crohn’s disease (CD). A causal relationship between inflammation and cancer has been proposed by Virchow, who hypothesized that malignant neoplasms are consequences of chronic inflammation (17). The reported risk varies widely among studies, but it is now established that patients with UC or CD show an increased prevalence of CRC (18,19).
Both IBD-associated and sporadic CRC are characterized by a dysplastic cancer sequence and multiple mutations must occur before a carcinoma develops (Figure 2) (20). When the CRC is sporadic, inflammation does not initiate tumorigenesis. Indeed, intratumoral immune cells are recruited after the tumor is formed and so, in this case, chronic inflammation does not precede but rather follows tumor development. In sporadic CRC, inflammation is thus more a consequence than a cause of tumor development. Unlike what is noted in sporadic CRC, chronic inflammation precedes CAC development (Figure 2).
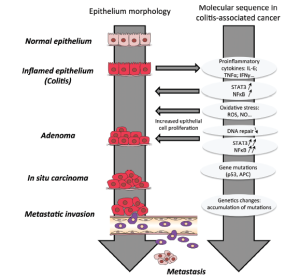
The pivotal role plays by NF-κB in colitis-associated colorectal cancer
It is becoming increasingly clear that cytokines and growth factors released during chronic inflammation may influence carcinogenesis. In IBD, chronic inflammation causes the release of cytokines, growth factors, proteases, and reactive oxygen species (ROS). These materials control the sequential recruitment of leukocytes, stimulate endothelial cells and fibroblasts to divide, and induce tissue remodeling. It has also been shown that infection with Helicobacter pylori induces active inflammation with infiltration of lymphocytes and macrophages/monocytes into the lamina propria of the mucosa of the human gastric antrum. Neutrophils and macrophages/monocytes produce ROS or reactive nitrogen species, which potentially damage DNA. Oxidative DNA damage in gastric cells is more prevalent in some genes than in others; the preferentially targeted genes notably include p53. Mutations in this protein are usually observed in both tumor cells and inflamed tissues (21). Cytokines released during inflammation may contribute to cancer development via activation of NF-κB.
NF-κB regulates the expression of various cytokines and modulates the inflammatory processes characteristic of IBD (22). Currently, several lines of evidence suggest that activation of NF-κB may play a crucial role in cancer development. Notably, it has been shown that NF-κB is aberrantly activated in 50% of CRC patients and those with colitis-associated tumors, and mouse studies have established that NF-κB plays a role in development of CAC (23,24). Further, NF-κB controls apoptosis, cell-cycle progression, cell proliferation, and differentiation. Activation of the IKK/NF-κB pathway is a key survival mechanism in a variety of cancer types. Numerous studies have found that NF-κB can block apoptosis by positively regulating the expression of anti-apoptosis proteins, such as inhibitors of the actions of apoptotic proteins. Notably, negative feedback loops exist between NF-κB pathway and that of various death-promoting proteins. Caspase-mediated cleavage of RelA and IKKβ can prevent NF-κB activation (25). Another mechanism whereby NF-κB may inhibit apoptosis is its ability to suppress prolonged activation of c-Jun N-terminal kinases (JNKs) and the accumulation of ROS. Indeed, suppression of NF-κB by ablation of RelA or IKKb causes persistent JNK induction by TNFα, resulting in cell death (26).
The role played by NF-κB in tumor development involves both classical and alternative pathways. Both Azoxymethane (AOM)/Dextran Sodium Sulfate (DSS) mouse models of CAC induction (27), and animal model carrying a conditional disruption of Ikkβ have been used to show that IKKβ-driven NF-κB activation within intestinal epithelial cells (IECs) is essential for development of colonic adenomas. The oncogenic role played by NF-κB in IECs appears to depend on anti-apoptotic functions of the protein, principally induction of Bcl-XL, which prevents apoptotic elimination of pre-malignant cells (23). In addition to this autonomous function in pre-malignant IECs, IKKβ-driven NF-κB activation contributes to CAC development when the process occurs in certain myeloid cells, most likely macrophages of the lamina propria. Activation of NF-κB in such cells stimulates the secretion of growth factors which, in turn, enhance proliferation of pre-malignant IECs.
Constitutive activation of the non-canonical NF-κB pathway in CRC has been reported in response to β-catenin accumulation (28). In carcinoma tissues with high levels of nuclear β-catenin, the phospho-p105 level was increased and the total p105 concentration decreased in comparison to the levels seen in normal tissue, indicating that the NF-κB pathway was activated in response to β-catenin.
Although NF-κB activation has been shown to be involved in CRC development, normally functioning NF-κB is essential for maintenance of epithelial cell homeostasis in the gut. Deregulation of NF-κB expression can cause chronic inflammation (29). In gut epithelial cells, NF-κB regulates epithelial integrity and interactions between the mucosal immune system and the gut microflora. IEC-specific inhibition of NF-κB synthesis via conditional ablation of NEMO (also termed IKKγ), or by conditional inactivation of both the IKKα and IKKβ subunits essential for NF-κB activation, results in the spontaneous development of severe chronic intestinal inflammation in mice. NF-κB deficiency caused colonic epithelial cells to enter apoptosis, impaired expression of antimicrobial peptides, and inhibited translocation of bacteria into the mucosa. NF-κB signaling maintains the host-microbiota homeostasis (29).
High levels of NF-κB proteins have been detected in epithelial cells and macrophages from patients with UC, CD, and unspecific colitis, providing evidence for constitutive NF-κB activation in such patients (30). Various pro-inflammatory stimuli induce NF-κB activation in different cell types; these stimuli include bacterial products interacting with Toll-like receptors, and/or cytokines (TNF-α and IL-1α). Blockage of such signaling has been shown to suppress experimental colitis (31). On the other hand, translocation of activated NF-κB into the nucleus induces the expression of cytokines such as TNF-α and IL-6, and chemokines, all of which contribute to development of inflammation-related tissue damage. Elevated IKK/NF-κB activity may cause aberrant upregulation of various tumorigenic proteins, adhesion proteins, chemokines, and inhibitors of apoptosis, all of which promote cell survival. Therefore, NF-κB may contribute to the development of colitis-associated CRC by sustaining an ongoing inflammatory process in the gut mucosa. Inactivation of the IκB kinase/NF-κB pathway at the site of inflammation, using mice that lack IKK only in intestinal epithelial cells, has been shown to attenuate formation of inflammation-associated tumors in the model AOM-DSS induced CAC (32).
The NF-κB pathway as a therapeutic target in colon cancer patients
The fact that the NF-κB signaling pathway plays pivotal roles in apoptosis, tumor promotion, and tumor maintenance, strongly suggests that inhibitors of this pathway would be powerful anti-cancer agents.
Thalidomide, a synthetic derivative of glutamate, has been shown to have both anti-inflammatory and anti-angiogenic effects in patients with several types of inflammatory diseases and cancers (33). This is attributable to the ability of thalidomide to suppress the DNA-binding activity of NF-κB. Moreover, using a human epithelial colon cancer cell line, thalidomide was shown to inhibit the IL-1β-induced IL-8 production subsequently to an inhibition of the IκBα degradation and NF-κB nuclear translocation (34).
Certain phytochemicals have beneficial effects in CRC patients. The most extensively investigated is curcumin, a yellow pigment from the turmeric plant (Curcuma longa). The pigment has been used for centuries to flavor and color foods, and as an anti-inflammatory medicine, in India and China (35). Extensive work has shown that curcumin exerts several pharmacological activities, including antioxidant, anticancer, and antiangiogenic effects. The anticancer properties of curcumin include inhibition of cancer cell proliferation in vitro, with enhancement of apoptosis, and anti-tumorigenesis effects in vivo. Many studies have shown that the inhibitory effect exerted by curcumin on the NF-κB pathway is involved in its anti-carcinogenesis and anti-angiogenesis properties. Curcumin suppresses NF-κB activation; downregulates expression of NF-κB-regulated genes; and inhibits cell proliferation, cell migration, and angiogenesis both in vitro and in vivo (36-41). Another compound derived from curcumin, termed C086, has recently been shown to be more potent than curcumin to inhibit cell growth and to downregulate NF-κB activity in both colon cancer cells and xenograft tumors (42).
The interaction between tumor and immune cells primarily controls overall tumor progression (or regression), the spread or induction of antitumor immune responses, and tumor rejection (43). Cytokines can act as inducers of the immune system via activation of T cells. Notably, a recent study found that the newly discovered cytokine IL-32γ, formerly termed natural killer (NK) cell transcript 4, can inhibit cancer cell growth. IL-32γ plays an important role in reducing colon cancer development via inhibition of NF-κB and STAT3 signaling, associated with reductions in the levels of pro-inflammatory cytokines (44).
Cancer cells express aberrant surface glycoproteins, including MUC1 and CD44, that have been suggested to play roles in cancer development by affecting tumor cell adhesion and signaling, and by reducing the effectiveness of immune surveillance. In tumor cells, CD43, a type I transmembrane protein featuring a mucin-like highly O-glycosylated extracellular domain, is suspected to reduce the efficiency of tumor/immune cell interactions. Laos et al. (45) showed that overexpression of CD43 inhibited NF-κB transcriptional activity and reduced the DNA binding of the transcriptional factor p65 in colon cancer cell lines (45). These data thus also suggest that targeting of NF-κB expression may be very effective to prevent CAC.
Gurova et al. (46) showed that quinacrine, first synthesized in 1931 and initially used as an antiprotozoal agent, upregulates p53 synthesis and inhibits activation of the NF-κB pathway in renal carcinoma cells. A more recent study using various carcinoma cell lines evaluated the possible role that quinacrine might play as a useful inhibitor of constitutive NF-κB activation. Inhibition of the NF-κB pathway by quinacrine was cytotoxic to human colon carcinoma cell lines in a p53 independent manner (47).
Gallotannin, a hydrolysable form of tannin found in the leaves and bark of many plants, inhibits the proliferation of various tumor cells, including those of colorectal carcinoma, prostate cancer, and acute myeloid leukemia, without any evident toxicity on normal cells. Gallotannin was first found to inhibit NF-κB-mediated transcription in lung epithelial cells (48), and treatment of human colon cancer cells caused cell-cycle arrest at the S phase; cells accumulated in the pre-G1 phase. The molecule inhibited NF-κB pathway action and its DNA-binding activity. In vivo, gallotannin suppressed tumor growth, decreased the levels of mRNAs encoding various proinflammatory mediators, and reduced the concentrations of proteins required for proliferation and angiogenesis (49).
NSAIDs exhibit antineoplastic activities in the colon (50,51). Large population-based studies have suggested that the relative risk of developing colon cancer is reduced by approximately 40-50% upon chronic use of NSAIDs such as aspirin. Moreover, the NSAID sulindac, commonly used to treat fever or pain resulting from inflammation-associated diseases such as rheumatoid arthritis, reduces the size and number of colonic polyps in familial adenomatous polyposis (FAP) patients. Several lines of evidence suggest that NF-κB is an NSAID target (52). Stimulation of NF-κB expression may be inhibited by various NSAIDs including aspirin, salicylate, and sulindac (53,54). Interestingly, more recent reports describe NF-κB activation by NSAIDs (55,56). Hence, it appears that NSAIDs can either inhibit or activate NF-κB. Nuclear translocation of NF-κB is responsible for aspirin-induced cell death (56). Thus, the data indicate that aspirin has a novel mechanism of action and the work will be of assistance in development of new chemopreventive agents for cancer treatment.
Dexamethasone, a glucocorticoid, is a potent NF-κB inhibitor, and clinical trials have been conducted to investigate the effectiveness of this agent in combination with other therapeutic drugs. The results suggest that dexamethasone may be useful to inhibit CRC metastasis to the liver (57).
The drugs described above potently inhibit NF-κB in vitro or in vivo or both, and some have already found clinical application. The NF-κB pathway is involved in many physiological functions. Thus, some adverse side-effects have, not surprisingly, been noted when the pathway is systemically inhibited. An ideal drug would target the site of required action and would be effective even in small concentrations.
Conclusions
Colorectal cancer is a common complication in IBD patients, and NF-κB is suspected to be the essential link between inflammation and carcinogenesis. Thus, proteins of the NF-κB pathway, or NF-κB per se, could be useful therapeutic targets in CAC patients. A considerable body of evidence from experiments with cells and whole animals indicates that NF-κB inhibitors may be potent if used as drugs to treat colon cancer. Although the expression of appropriate levels of NF-κB is essential if normal cell proliferation is to be maintained, constitutive NF-κB activation is likely involved in the enhanced growth properties characteristic of many cancers. Work on inhibition of the NF-κB pathway in cancer patients is at an early stage. However, such approaches offer the promise of improving cancer chemotherapy and reducing production of the abnormal cytokines that may stimulate the growth of certain tumors.
A problem is that the NF-κB signaling pathway is very complex and is involved in many physiological functions. Thus, wholesale inhibition of the pathway may have unanticipated adverse effects. An ideal NF-κB inhibitor should prevent NF-κB activation in the absence of any adverse effects on other signaling pathways and should not cause long-term immune suppression.
Thus, the principal challenge to be dealt with before treatment of CAC patients via inhibition of the NF-κB pathway is to specifically target the site of inflammation and/or cancer initiation by delivering drugs in dedicated carriers. Nanotechnology may aid in therapeutic targeting of CAC. Nanoparticles are 1-100 nm in diameter and can transport drugs within the body. Thus, such particles may serve as carrier molecules delivering drugs to sites of inflammation. This drug delivery system is versatile and has been used to overcome physiological barriers to the targeting of some NF-κB inhibitors to the colon, the specific site of inflammation (58). With the aid of nanoparticles, NF-κB inhibitors can be used at very small concentrations because the drugs are delivered to restricted areas. Such technology would reduce or eliminate the adverse side-effects currently noted after systemic administration of anti-inflammatory drugs to patients with CRC or colitis.
Acknowledgements
This work was supported by grants from the Department of Veterans Affairs and the National Institutes of Health of Diabetes and Digestive and Kidney by the grant RO1-DK-071594 (to D.M).
We dedicate this article to the memory of Dr. Shanthi V. Sitaraman, a brilliant scientist, dedicated physician, passionate humanitarian and dearest friend.
Disclosure: The authors declare no conflict of interest.
References
- Jemal A, Bray F, Center MM, et al. Global cancer statistics. CA Cancer J Clin 2011;61:69-90.
- Ligibel J. Lifestyle factors in cancer survivorship. J Clin Oncol 2012;30:3697-704.
- Wei EK, Giovannucci E, Wu K, et al. Comparison of risk factors for colon and rectal cancer. Int J Cancer 2004;108:433-42.
- Hagemann T, Balkwill F, Lawrence T. Inflammation and cancer: a double-edged sword. Cancer Cell 2007;12:300-1.
- Mantovani A, Allavena P, Sica A, et al. Cancer-related inflammation. Nature 2008;454:436-44.
- Chan AT, Ogino S, Fuchs CS. Aspirin and the risk of colorectal cancer in relation to the expression of COX-2. N Engl J Med 2007;356:2131-42.
- Flossmann E, Rothwell PM, British Doctors Aspirin Trial and the UK-TIA Aspirin Trial. Effect of aspirin on long-term risk of colorectal cancer: consistent evidence from randomised and observational studies. Lancet 2007;369:1603-13.
- Baeuerle PA, Henkel T. Function and activation of NF-kappa B in the immune system. Annu Rev Immunol 1994;12:141-79.
- Barnes PJ, Karin M. Nuclear factor-kappaB: a pivotal transcription factor in chronic inflammatory diseases. N Engl J Med 1997;336:1066-71.
- Karin M, Lin A. NF-kappaB at the crossroads of life and death. Nat Immunol 2002;3:221-7.
- Karin M. Nuclear factor-kappaB in cancer development and progression. Nature 2006;441:431-6.
- Rayet B, Gélinas C. Aberrant rel/nfkb genes and activity in human cancer. Oncogene 1999;18:6938-47.
- Wang CY, Cusack JC Jr, Liu R, et al. Control of inducible chemoresistance: enhanced anti-tumor therapy through increased apoptosis by inhibition of NF-kappaB. Nat Med 1999;5:412-7.
- Perkins ND. Integrating cell-signalling pathways with NF-kappaB and IKK function. Nat Rev Mol Cell Biol 2007;8:49-62.
- Hayden MS, Ghosh S. Signaling to NF-kappaB. Genes Dev 2004;18:2195-224.
- Karin M, Ben-Neriah Y. Phosphorylation meets ubiquitination: the control of NF-[kappa]B activity. Annu Rev Immunol 2000;18:621-63.
- Balkwill F, Mantovani A. Inflammation and cancer: back to Virchow? Lancet 2001;357:539-45.
- Askling J, Dickman PW, Karlén P, et al. Colorectal cancer rates among first-degree relatives of patients with inflammatory bowel disease: a population-based cohort study. Lancet 2001;357:262-6.
- Jess T, Rungoe C, Peyrin-Biroulet L. Risk of colorectal cancer in patients with ulcerative colitis: a meta-analysis of population-based cohort studies. Clin Gastroenterol Hepatol 2012;10:639-45.
- Fearon ER, Vogelstein B. A genetic model for colorectal tumorigenesis. Cell 1990;61:759-67.
- Choi J, Yoon SH, Kim JE, et al. Gene-specific oxidative DNA damage in Helicobacter pylori-infected human gastric mucosa. Int J Cancer 2002;99:485-90.
- Schottelius AJ, Dinter H. Cytokines, NF-kappaB, microenvironment, intestinal inflammation and cancer. Cancer Treat Res 2006;130:67-87.
- Greten FR, Eckmann L, Greten TF, et al. IKKbeta links inflammation and tumorigenesis in a mouse model of colitis-associated cancer. Cell 2004;118:285-96.
- Kojima M, Morisaki T, Sasaki N, et al. Increased nuclear factor-kB activation in human colorectal carcinoma and its correlation with tumor progression. Anticancer Res 2004;24:675-81.
- Tang G, Yang J, Minemoto Y, et al. Blocking caspase-3-mediated proteolysis of IKKbeta suppresses TNF-alpha-induced apoptosis. Mol Cell 2001;8:1005-16.
- Papa S, Zazzeroni F, Pham CG, et al. Linking JNK signaling to NF-kappaB: a key to survival. J Cell Sci 2004;117:5197-208.
- Okayasu I, Ohkusa T, Kajiura K, et al. Promotion of colorectal neoplasia in experimental murine ulcerative colitis. Gut 1996;39:87-92.
- Lauscher JC, Gröne J, Dullat S, et al. Association between activation of atypical NF-kappaB1 p105 signaling pathway and nuclear beta-catenin accumulation in colorectal carcinoma. Mol Carcinog 2010;49:121-9.
- Nenci A, Becker C, Wullaert A, et al. Epithelial NEMO links innate immunity to chronic intestinal inflammation. Nature 2007;446:557-61.
- Rogler G, Brand K, Vogl D, et al. Nuclear factor kappaB is activated in macrophages and epithelial cells of inflamed intestinal mucosa. Gastroenterology 1998;115:357-69.
- Senftleben U, Li ZW, Baud V, et al. IKKbeta is essential for protecting T cells from TNFalpha-induced apoptosis. Immunity 2001;14:217-30.
- Greten FR, Karin M. The IKK/NF-kappaB activation pathway-a target for prevention and treatment of cancer. Cancer Lett 2004;206:193-9.
- Stephens TD, Fillmore BJ. Hypothesis: thalidomide embryopathy-proposed mechanism of action. Teratology 2000;61:189-95.
- Jin SH, Kim TI, Han DS, et al. Thalidomide suppresses the interleukin 1beta-induced NFkappaB signaling pathway in colon cancer cells. Ann N Y Acad Sci 2002;973:414-8.
- Surh YJ. Cancer chemoprevention with dietary phytochemicals. Nat Rev Cancer 2003;3:768-80.
- Aggarwal S, Ichikawa H, Takada Y, et al. Curcumin (diferuloylmethane) down-regulates expression of cell proliferation and antiapoptotic and metastatic gene products through suppression of IkappaBalpha kinase and Akt activation. Mol Pharmacol 2006;69:195-206.
- Anto RJ, Mukhopadhyay A, Denning K, et al. Curcumin (diferuloylmethane) induces apoptosis through activation of caspase-8, BID cleavage and cytochrome c release: its suppression by ectopic expression of Bcl-2 and Bcl-xl. Carcinogenesis 2002;23:143-50.
- Mukhopadhyay A, Banerjee S, Stafford LJ, et al. Curcumin-induced suppression of cell proliferation correlates with down-regulation of cyclin D1 expression and CDK4-mediated retinoblastoma protein phosphorylation. Oncogene 2002;21:8852-61.
- Sandur SK, Deorukhkar A, Pandey MK, et al. Curcumin modulates the radiosensitivity of colorectal cancer cells by suppressing constitutive and inducible NF-kappaB activity. Int J Radiat Oncol Biol Phys 2009;75:534-42.
- Singh S, Aggarwal BB. Activation of transcription factor NF-kappa B is suppressed by curcumin (diferuloylmethane) [corrected]. J Biol Chem 1995;270:24995-5000.
- Su CC, Chen GW, Lin JG, et al. Curcumin inhibits cell migration of human colon cancer colo 205 cells through the inhibition of nuclear factor kappa B /p65 and down-regulates cyclooxygenase-2 and matrix metalloproteinase-2 expressions. Anticancer Res 2006;26:1281-8.
- Chen C, Liu Y, Chen Y, et al. C086, a novel analog of curcumin, induces growth inhibition and down-regulation of NFκB in colon cancer cells and xenograft tumors. Cancer Biol Ther 2011;12:797-807.
- Shurin MR, Shurin GV, Lokshin A, et al. Intratumoral cytokines/chemokines/growth factors and tumor infiltrating dendritic cells: friends or enemies? Cancer Metastasis Rev 2006;25:333-56.
- Oh JH, Cho MC, Kim JH, et al. IL-32γ inhibits cancer cell growth through inactivation of NF-κB and STAT3 signals. Oncogene 2011;30:3345-59.
- Laos S, Baeckström D, Hansson GC. Inhibition of NF-kappaB activation and chemokine expression by the leukocyte glycoprotein, CD43, in colon cancer cells. Int J Oncol 2006;28:695-704.
- Gurova KV, Hill JE, Guo C, et al. Small molecules that reactivate p53 in renal cell carcinoma reveal a NF-kappaB-dependent mechanism of p53 suppression in tumors. Proc Natl Acad Sci U S A 2005;102:17448-53.
- Jani TS, DeVecchio J, Mazumdar T, et al. Inhibition of NF-kappaB signaling by quinacrine is cytotoxic to human colon carcinoma cell lines and is synergistic in combination with tumor necrosis factor-related apoptosis-inducing ligand (TRAIL) or oxaliplatin. J Biol Chem 2010;285:19162-72.
- Erdèlyi K, Kiss A, Bakondi E, et al. Gallotannin inhibits the expression of chemokines and inflammatory cytokines in A549 cells. Mol Pharmacol 2005;68:895-904.
- Al-Halabi R, Bou Chedid M, Abou Merhi R, et al. Gallotannin inhibits NFĸB signaling and growth of human colon cancer xenografts. Cancer Biol Ther 2011;12:59-68.
- Piazza GA, Rahm AK, Finn TS, et al. Apoptosis primarily accounts for the growth-inhibitory properties of sulindac metabolites and involves a mechanism that is independent of cyclooxygenase inhibition, cell cycle arrest, and p53 induction. Cancer Res 1997;57:2452-9.
- Shiff SJ, Koutsos MI, Qiao L, et al. Nonsteroidal antiinflammatory drugs inhibit the proliferation of colon adenocarcinoma cells: effects on cell cycle and apoptosis. Exp Cell Res 1996;222:179-88.
- Shiff SJ, Rigas B. Aspirin for cancer. Nat Med 1999;5:1348-9.
- Kopp E, Ghosh S. Inhibition of NF-kappa B by sodium salicylate and aspirin. Science 1994;265:956-9.
- Yamamoto Y, Yin MJ, Lin KM, et al. Sulindac inhibits activation of the NF-kappaB pathway. J Biol Chem 1999;274:27307-14.
- Moalic-Juge S, Liagre B, Duval R, et al. The anti-apoptotic property of NS-398 at high dose can be mediated in part through NF-kappaB activation, hsp70 induction and a decrease in caspase-3 activity in human osteosarcoma cells. Int J Oncol 2002;20:1255-62.
- Stark LA, Din FV, Zwacka RM, et al. Aspirin-induced activation of the NF-kappaB signaling pathway: a novel mechanism for aspirin-mediated apoptosis in colon cancer cells. FASEB J 2001;15:1273-5.
- Kemeny N, Capanu M, D’Angelica M, et al. Phase I trial of adjuvant hepatic arterial infusion (HAI) with floxuridine (FUDR) and dexamethasone plus systemic oxaliplatin, 5-fluorouracil and leucovorin in patients with resected liver metastases from colorectal cancer. Ann Oncol 2009;20:1236-41.
- Laroui H, Dalmasso G, Nguyen HT, et al. Drug-loaded nanoparticles targeted to the colon with polysaccharide hydrogel reduce colitis in a mouse model. Gastroenterology 2010;138:843-53.e1-2.