The ErbB2 receptor in gastric cancer: the quick-change artist
Introduction
Gastric cancer (GC) is the fourth most common cancer and the second leading cause of mortality worldwide (1,2) with a case fatality rate of 75%. It is still one of the most important malignant diseases with significant geographical, ethnic and socioeconomic differences in distribution. Most of the GCs (90%) are sporadic. Familial clustering is observed only in 10% of cases and, among these, only 1-3% of GCs are considered hereditary with the inclusion of hereditary diffuse gastric cancer (HDGC) and the gastric adenocarcinoma and proximal polyposis of the stomach (GAPPS) (3-6). Unfortunately, most GC are diagnosed in advanced or metastatic stage, and palliative chemotherapy remains the only treatment option, while the surgical resection is the mainstay of treatment for patients at early-stage cancer but the survival rate of patients with advanced respectable GC or gastroesophageal junction (GEJ) cancer, remains poor.
Despite the significant improvements of modern technology that contributed to shed light on the molecular bases sustaining development, invasion and metastasis, GC remains a major diagnostic and therapeutic challenge. This is due to the wide heterogeneity of cell population with a variety of genetic lesions in the same neoplastic foci, but it is also due to the existence of at least five cancer subtypes with different molecular pathogenesis and clinicopathological profiles, classified by the World Health Organization as: papillary, tubular and mucinous adenocarcinomas, mixed carcinomas and poorly cohesive carcinomas, with or without culprits of ring cells (7). More recent work by Lei et al. provided a further subdivision of GC according to their ability to respond to PI3-K inhibitors and 5-fluoracile (5-FU), identifying three subset of GCs described as “mesenchymal”, “proliferative” and “metabolic”, distinguishable for histological phenotypes and sustained by different patterns of mutated gene expression (8,9). For all these reasons the molecular pathogenesis of GC is complex, and this reflects the limited success in the treatment of this neoplasia. Nevertheless, in sporadic GC the more than 37% of gene alteration are found in the pathways of tyrosine kinase receptors (RTK) and are potentially drug-gable. Among the growth factor receptors, the EGFR family supplies most of the oncogenic signals through the overexpression of ErbB2 (15% of GCs), EGFR (40% of GCs) and through the expression ErbB3 documented in 62% of GC (10,11). FGFR2 and mesenchymal epithelial transition (MET) receptor amplification, phos¬phatidylinositol 3-kinase (PI3K), PTEN and KRAS mutations, contribute to amplify the signals promoting cell cycle and suppressing the apoptotic pathways (12,13) (Table 1).
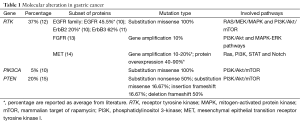
Full table
Unlike in breast cancer, the studies in GC have produced conflicting findings regarding the prognostic relevance of ErbB2. However, most of the reports indicate the overexpression of ErbB2 and any of the ErbB receptors, as variably associated to aggressive phenotype and poor survival of gastric adenocarcinomas. In particular, while EGFR and ErbB2 overexpression can valuably predict poor outcomes in GC patients, high levels of ErbB3 that are commonly detected in advanced GC, have been associated with tumor resistance to EGFR- and ErbB2-targeted agents (16). Yet, despite any dispute over the prognostic properties of overexpressed ErbB2 in GC, there is more consensus regarding the importance of this receptor as a therapeutic target, fact that justifies the continue effort to develop and test novel biological agents in pre-clinical and clinical settings (17-19). The usefulness of ErbB2 targeted therapy has been first demonstrated by the relative success of ToGA trial, still considered as a landmark in the treatment of GC. ToGA trial reported a modest although significant prolongation of progression-free survival (PFS) in patients with ErbB2-overexpressing metastatic GC or GEJ adenocarcinoma treated with trastuzumab, a therapeutic antibody anti-ErbB2, in combination with cisplatin and a fluoropyrimidine (either capecitabine or 5-FU), as compared to chemotherapy alone (20). Results indicate that, while the early responses to anti-receptor therapies can be successfully due to uncoupling the links between cell surface receptors and gene expression, the progression of the disease is characterized by the appearance of vicarious or overlapping pathways for tumor growth and survival that decrease or abrogate the efficacy of the treatment over a short period of time.
Considering the increasing incidence of gastric and GEJ adenocarcinomas typically displaying high ErbB2 expression levels, there is the urgent need to elucidate responses and adaptive mechanisms utilized by cancer gastric cells during tumor progression, but more importantly it is necessary to determine the optimal use of anti ErbB2 targeted therapies and identify rational strategies to overcome the acquired resistance.
In this review we attempt to analyze the mechanisms sustaining the activation of alternative oncogenic pathways potentially responsible for the occurrence of primary and secondary resistance to chemotherapy or anti-ErbB2 therapies, indicating alternative views for the identification of strategies to successfully target GC with suitable ErbB2-targeted compounds.
The ErbB2 receptor in the EGFR/ErbB signaling network
The EGFR receptor family is made up of four distinct members: ErbB1 (EGFR/HER1), ErbB2 (HER2/neu), ErbB3 (HER3) and ErbB4 (HER4) (21). These receptors are evolutionary conserved and contain functional redundancy to ensure signaling is maintained and effective. They are involved in the execution of a number of developmental programs that requires generation of fine-tuned signals spatially and temporally regulated by a wealth of ligands necessary to elicit specific cellular responses. The ErbB network comprises of a ligand-dependent aggregation of active receptors in homo- and heterodimeric conformation, whose dynamics are regulated in accordance to a hierarchical model of inter-receptor interactions.
In principle, the mechanism of activation of the ErbB receptors relies on the availability in the extracellular environment of ligands differently able to drive the formation of homo- or hetero-dimers according to their own binding specificities. However, ErbB2 has no known ligands and ErbB3 can intercept growth factor ligands but contains much lower intrinsic kinase activity. Indeed, the two impaired receptors, either in their ligand-binding ability (ErbB2), or in the kinase activation domain (ErbB3), need an interdependent and mutual interaction to supply to their own limited abilities. Together, they trigger and propagate signals leading to the activation of the Ras/Raf/mitogen-activated protein kinase (MAPK) and PI3K/Akt/mammalian target of rapamycin (mTOR) downstream signaling pathways, and for this ErbB2/ErbB3 are considered the most potent signaling complex in terms of growth and transformation (22,23).
The ErbB receptors can be variably stimulated by a plethora of growth factor ligands encoded by more than 20 genes (24). Seven of these ligands bind to the EGFR and nine to ErbB4, with HB-EGF, epiregulin and betacellulin shared. ErbB3 displays more selective interactions, being able to bind Nrg1 and Nrg2 ligands (25-28) (Figure 1A). Notably, the tridimensional structure of the ErbB2 extracellular domain (ECD) confers unique features to this receptor that, at high receptor density, is able to form active dimers in absence of ligands and of any other receptor partner. The ErbB receptors can be also activated by non-physiological stimuli (e.g., oxidative stress, UV, and γ-irradiation) or by other RTKs (notably MET, IGF-1R, or TRK-B). In this network of highly organized interactions the receptors contribute to specific signaling responses by recruiting enzymes, adaptors and scaffolds proteins through a variety of recognition signals that are specific for the different receptor types.

In general, quality and quantity of the signaling dimers depend on the stoichiometry among the all ErbB receptors expressed and on the availability of ligands that, in virtue of their affinities and binding specificities, can variably orient the hierarchy of receptor dimer formation. Thus, combination of expression level variability and inter-receptor interactions account for a wide repertoire of signaling options. This complexity become particularly evident in tumor cells, where different co-expression profiles of ErbB1-4 and EGF-like growth factors define different cancer types and subtypes, and sustain oncogenic programs through the activation of specific downstream signaling cascades (29,30).
In relative paucity of ErbB receptors on the surface of cancer cells, highly expressed ErbB2 tend to aggregate to form active dimers able to signal even in absence of ligand. On the other hand, the availability of significant amount of other ErbB receptors allows for the distribution of ErbB2 in hetero-dimeric conformation, able to aggregate according to ligand binding specificities. This modality of activation for the ErbB receptors implies that ErbB2 overexpression might be not exhaustive to define subset of aggressive tumors, but should also be related to the expression of ErbB3 and EGFR, particularly prone to dimerize with ErbB2.
More complexity is added by the dynamic redistribution of active dimers containing ErbB2 during tumor progression per se, or in response to anti-RTK treatments, when cancer cells become resistant to mono-drug therapies. In all cases, cancer cells redefine by time quantities and stoichiometry of the active dimers, with significant changes in the quality and potency of downstream signaling events promoting proliferation, migration or anti-apoptotic responses. Acceptance of the “dynamic hierarchical model for inter-receptor interaction” implies a reinterpretation of the overexpression of ErbB2 in cancer cells, overall in light of the possibility to choose different therapeutic molecules, kinase inhibitors and monoclonal antibodies (mAbs) to specifically target ErbB2 and its numerous RTKs dimer partners. The major challenge is to overcome the reprogramming of the RTK network induced by drug resistance, fact that can be obtained by the combinatorial use of drugs diversely directed to target all the dimers containing ErbB2.
Activation of ErbB3: a mechanism to resist?
The limited efficacy of anti-ErbB2 therapies suggests the presence of alternative pathways of escape for cancer cells. ErbB3 is now emerging as a key player in the establishment of malignancy cancer progression and, above all, in the establishment of resistance to therapies (31). Despite the presence of an impaired kinase domain in its intra-cytoplasmic region, ErbB3 can be trans-phosphorylated by lateral signaling from other RTKs of the same or other families to promote activation of the PI3K/Akt signaling pathway. From mechanistic standpoint, the six tyrosine residues in its cytoplasmic tail when phosphorylated become high affinity binding sites for the regulatory subunit of PI3K. Recruitment of PI3K activity sustains metabolic pathways regulating cells proliferation, tumor size, and resistance to apoptosis and is required for the early steps that regulates cell motility and invasion of metastatic cells. Therefore, the high expression of ErbB3 has been associated with tumor progression, depth of invasion, lymph node metastasis, stage of GC and, more significantly, with a worse overall survival (OS) (16,19,32). Notably, the higher levels of ErbB2 and ErbB3 have been detected in stage III-IV gastric adenocarcinoma compared to in stage I-II disease (22-24.0% vs. 5.8-7.7%, P<0.05) (33).
The mechanisms involved in the feedback regulation of the PI3K/Akt axis at the ErbB3 receptor level are numerous and vary from the down-regulation of suppressive miRNA, to a FOXO-dependent induction of ErbB3 transcripts; from the RAS-dependent up-regulation of the NRG-1 ligand regulating the phosphorylation of ErbB3, to an inefficient sorting of the receptor to the degradation pathway when coupled with ErbB2 (34-37). All of these conditions amplify the ErbB3-dependent PI3K/Akt signals and lead cells to proliferate.
On the other hand, many studies indicate that up-regulation of ErbB3 may promote resistance to a number of inhibitors designed to antagonize the PI3K activation. Preliminary data sustain also the hypothesis that the ErbB3 receptor is involved in the activation of an early feedback survival loop in cells exposed to BRAF and/or MEK inhibitors, with a mechanism leading to enhanced production of NRG-1 (unpublished data).
In the last years, evidence has begun to emerge that ErbB3 can be activated by mutations able to confer transforming capabilities in vivo and in vitro. The majority of these mutations identified in human tumors cluster in the ECD region, with few of them in the kinase domain and in the intracellular tail of ErbB3 (38,39). Single amino acid substitutions found in seven hot spot positions in the ECD seem to confer an untethered and open conformation to ErbB3 that is primed for dimerization and activation (39). Jaiswal and colleagues found recurrent mutations in the ErbB3 coding region in 12% and 11% of gastric and colon cancers (37). Notably, these mutations remove the need for ErbB3 of the lateral phosphorylation from active kinases and therefore, it remains relevant to successfully target the ErbB3 mutants, to consider drugs that inhibit dimerization or that inhibit the kinase activity of the dimerization partner.
These evidences have triggered major efforts towards the development of anti-ErbB3 therapies, and in particular of anti-ErbB3 mAbs because this receptor is devoid of strong intrinsic kinase activity.
The hepatocyte growth factor (HGF)/mesenchymal epithelial transition (MET) pathway
Recent studies have revealed that the heterogeneous expression of multiple RTKs throughout the tumor is a principal mechanism of resistance. In particular, targeted inhibition of a single receptor is ineffective, and this is apparently due to the up-regulation of other receptors within the same family, or to the engagement of alternative pathways mediated by the ligand stimulation of other unrelated RTKs (40,41). Among these, the signaling of the hepatocyte growth factor receptor (MET-HGFr) may play important roles.
Upon HGF stimulation, MET induces several biological responses including cell invasion, proliferation and anchorage independent growth that collectively promote tumor invasiveness. Those programs are sustained by the activation of multiple signal transduction pathways, including Ras, PI3K, STAT and Notch pathway, this latter through the transcriptional activation of Delta ligand.
From the very early studies, MET expression has been recognized as an important poor prognostic marker in GC (14,42). A total of 15% of GC patients (between 10% and 20% in the literature) harbor c-MET gene amplification and 40% to 90% have protein overexpression. Both MET overexpres¬sion and gene alterations have been shown to significantly correlate with the depth of tumor invasion, metastatic behavior and poor prognosis, providing evidence for the key role of c-MET in GC and a rationale for the development of MET inhibitors (43-47).
Besides the canonical way of activation by natural ligands, Met signaling is also sustained by a bidirectional crosstalk with the EGFR: while EGFR inhibition reduces basal MET phosphorylation (48,49), MET inhibition reduces basal phosphorylation of EGFR, ErbB2 and ErbB3 (41,50). In MET overexpressing cells, MET triggers proliferation and anti-apoptotic signals through ErbB3 to sustain cell PI3K/Akt survival despite EGFR inhibition (41). The mutual interaction existing between the EGFR family and MET is also supported by observations that patients with GC with constitutive activation of the ErbB receptors responded poorly to MET inhibition, which indicates that activation of the MET/HGF signaling pathway is one of the mechanisms of resistance to EGFR/ErbB2 inhibitors (51). Interestingly enough, EGFR, MET and ErbB2 gene amplification are mutually exclusive events in GC (52).
All these evidences provide the rational for therapeutic actions aimed to target both the ErbB2 and MET receptors that, in GC subjected to single therapy against either one of the receptor, may link their own downstream signaling pathways, to become resistant.
PI3K/Akt/PTEN
The PI3K/Akt pathway is one of the main downstream signaling pathways of the ErbB receptors and MET, and is altered in a variety of human tumor types, including GC. Preclinical work has demonstrated that both PI3K mutations and PTEN (phosphate and tensin homolog) inactivation cause amplification of the downstream PI3K/Akt signals, in absence of ligand/receptor stimulation.
The PI3KCA gene encodes for the catalytic subunit p110α of the PI3K heterodimeric complex. Its gene amplification is commonly detected in advanced gastric cancer (aGC) patients with frequencies close to 67%, while activating mutations have been described in 5% to 7.1% of GC (53,54). Occurrence of these mutations appears to be a late event in gastric carcinogenesis, because their frequency is higher in T4 cancers (21.4%) than in T2 cancers (6.4%) (55). It is consistent with the role that PIK3CA and PI3K signaling have in acquired resistance to upstream inhibitors, including trastuzumab and pertuzumab. Notably, these agents can exert their antitumor effects in ErbB2 overexpressing cells only in presence of a normal PI3K pathway.
Functional inactivation of the tumor suppressor gene PTEN is a dynamic process during the course of cancer progression and has been observed in almost 20% of the GC patients. Mechanisms involved in PTEN deficiency are mostly related to gene mutations, loss of heterozygosity (LOH), promoter hyper-methylation and miRNA-mediated regulation. PTEN deficiency can be used as an indicator to diagnose the pathological state of GC but most importantly, when evaluated in combination with ErbB2 expression, identifies subsets of malignant phenotypes with more aggressive proliferative culprits (15). Furthermore, recent work of Zhang and colleagues identified higher prevalence of PTEN deficiency in patients resistant to trastuzumab or lapatinib and with shorter PFS, thus qualifying PTEN as an important predictive marker for early resistance to ErbB2 inhibitor treatment in GC patients (56).
Dual blockade anti-ErbB2 therapy
Trastuzumab and pertuzumab are two humanized recombinant antibodies currently employed to target the ErbB2 receptor in a variety of cancers (Figure 1B,C). These two mAbs have different but complementary mechanisms of action depending on their own ability to bind to ErbB2 at different epitopes. Whereas trastuzumab binds to subdomain IV of ErbB2 to inhibit ligand-independent signaling, pertuzumab sterically blocks a binding pocket for ErbB2 dimerization with other ErbB receptors, with the consequence to block the ligand-activated signaling from EGFR/ErbB2 and, particularly, from ErbB2/ErbB3 dimers at his source (57-59). In this case, the primary binding of pertuzumab to the subdomain II of the ErbB2 ECD and to a lesser extent with the domain I blocks the formation of heterodimers, but does not interfere with the ability of ErbB2 to form homo-dimers, thus leaving intact their abilities to elicit oncogenic signals (59,60). By contrast, binding of trastuzumab to ErbB2 results in the inhibition of receptor homo-dimerization without interfering with its hetero-dimerization with ligand-activated EGFR or ErbB3 (61,62). Beyond the inhibition of signal transduction through ErbB2 homo-dimers, trastuzumab inhibits the shedding of the ECD of ErbB2, which produces active truncated p95-ErbB2, suppresses tumor angiogenesis, inhibits DNA damage repair, while both trastuzumab and pertuzumab can elicit an antibody-dependent cell-mediated cytotoxicity.
The abilities of both the antibodies have been validated in several clinical trials, but unfortunately not all the patients with tumors overexpress ErbB2 respond to trastuzumab, and the majority of patients who achieve an initial response to trastuzumab-based regimens develop resistance within 1 year. One possible explanation is that the use of one single antibody may induce by time changes in the kinetics of dimer formation, with ErbB2 shifting from homodimers to an ErbB2/ErbB3 heterodimeric aggregation, with consequent variation in the balance between MAPK and PI3K pathways. This reprogramming of the signaling output is the first mean for adjustment of cancer cells to escape from a single drug administration. These concepts have been carefully evaluated by Goltsov and colleagues that used combination of experimental and computational models to calculate the inhibition of pAkt and pERK in cancer cells treated with pertuzumab and trastuzumab, alone or in combination (63). They found that trastuzumab alone inhibits pERK when ErbB2 is in homodimeric conformation (high ErbB2 and low ErbB3 expression), but has no effects on the PI3K signaling on the same cells. They also proved that effectiveness of trastuzumab on pERK inhibition decreases in high density of ErbB2/ErbB3 dimers, since most of the ErbB2 receptors signal with ErbB3. By contrast, pertuzumab is effective to down-regulate both pAkt and pERK in cells expressing high density of ErbB2/ErbB3, while it fails to inhibit pERK because it does not block ErbB2 homo-dimerization (Figure 1). The combination of the two treatments effectively inhibits pERK activity both high ErbB2 and ErbB2/ErbB3 compositions, while the effects of either one mono-therapy depends on ErbB2/ErbB3 dimerization kinetics that are under the strict control of ErbB2 and ErbB3 expression levels. In this context, the major role is plaid by compensatory shift in ErbB3 activation and up-regulation, it have been observed as a consequence of cell reprogramming following suboptimal inhibition by tyrosine kinase inhibitors (TKIs), or resistance to single drug therapy (64).
The concept of dynamic reprogramming of the ErbB dimers containing ErbB2 in cancer cells subjected to mono-therapy is supported by in vitro and in vivo preclinical studies. Targeting of the ErbB2 receptor with the simultaneous administration of trastuzumab and pertuzumab caused stronger growth inhibition of SKOV3 tumor xenograft, providing a more efficient blockade of the ErbB2 signaling pathway, than either antibody alone (65-68). Their use in combination in clinical settings has been successfully tested and consolidated in the Cleopatra study where patients with ErbB2-positive metastatic breast cancer treated with pertuzumab, trastuzumab and docetaxel significantly improved progression-free and OS compared with placebo, trastuzumab and docetaxel (69).
More recently, a phase IIa trial conducted to ascertain pharmacokinetics and safety in ErbB2-positive aGC patient treated with pertuzumab and trastuzumab in combination with capecitabine and cisplatin sustains the feasibility of a dual blockade anti-ErbB2 therapy with pertuzumab plus trastuzumab (70). Preliminary data from the exploratory efficacy analysis show that those patients treated with the combination achieved high rates of partial response (86% in Arm A and 55% in Arm B) or stable disease (SD) (14% in Arm A and 27% in Arm B) at the end of cycle 6. We are waiting for the result of double-blind, placebo-controlled, randomized, multicenter, international, parallel arm phase III study will evaluate the efficacy and safety of pertuzumab in combination with trastuzumab, fluoropyrimidine and cisplatin as first-line treatment in ErbB2-positive aGC or metastatic GEJ (Clinical Trials gov. NCT01774786).
Novel agents and promising molecules
When the patients acquire resistance to trastuzumab, what to do?
The molecular mechanisms underlying trastuzumab resistance in GC are complex, and intratumoral heterogeneity of this tumor certainly contributes to resistance. In this regard, others classes of targeted drugs, including TKIs, such as Lapatinib and Dacomitinib, mTOR pathway inhibitors, such as Everolimus, have also been investigated and are potentially useful in clinics.
Lapatinib, an oral tyrosine kinase inhibitor of EGFR and ErbB2, has also been tested in ErbB2-positive GC. Although in preclinical studies Lapatinib was shown to display synergistic effects with trastuzumab in human ErbB2 positive gastric cells, in vitro and in vivo, the randomized Phase III TRIO-013/Logic Trial failed to show significant improvement in the OS when combined with chemotherapy for first-line (71) or second-line treatment (72) of ErbB2-positive aGC, indicating again, either the existence of alternative drug-resistant pathways of escape from ErbB2-targeted therapies, or the induction of genetic changes sustaining resistance.
Dacomitinib (PF-00299804) is a second-generation irreversible pan-ErbB receptor tyrosine kinase inhibitor (selectively inhibiting EGFR, ErbB2, and ErbB4) under clinical development. The combination of Dacomitinib with chemotherapeutic agents (such as 5-FU and cisplatin) or targeted agents (such as trastuzumab) showed a synergistic effect. However, a clinical phase II study in ErbB2 positive patients with aGC, where Dacomitinib was given as monotherapy, showed a response rate of only 7.4% and an OS of 7.1 months.
Afatinib (Giotrif, Boehringer Ingelheim) is another oral irreversible pan-ErbB TKI, recently approved by FDA for first-line treatment of patients with metastatic NSCLC with EGFR exon 19 deletions or exon 21 substitution mutations. In GC, Afatinib has demonstrated antitumor activity in a ErbB2 positive xenograft mouse model and based on data from clinical phase II study in esophagogastric (EG) cancer patients, the investigators concluded that single agent afatinib showed clinical efficacy in patients with trastuzumab refractory EG cancer.
Crizotinib and Foretinib are c-Met inhibitors, in two small phase I/II studies: two patients harboring MET amplification were treated with crizotinib and presented tumor shrinkage (−30% and −16%) and experienced progression after 3.7 and 3.5 months. Shah et al. reported 67 aGC patients who were treated with Foretinib irrespective of c-Met status. Best response was SD in 10 (23%) patients receiving intermittent dosing and 5 (20%) receiving daily dosing; SD duration was 1.9-7.2 months (median 3.2 months) (73).
T-DM1 is an antibody-drug conjugate in which trastuzumab is conjugated to a cytotoxic compound, emtansine (DM1). T-DM1 combines the mode of action of trastuzumab with the targeted delivery of a potent cytotoxic (74,75). Upon binding of the trastuzumab moiety to ErbB2, T-DM1 is internalized into the tumor cell, releasing the DM1 moiety, which inhibits microtubules. A trial is now underway to examine the efficacy and safety of T-DM1 compared with standard taxane therapy in patients with ErbB2-positive GC (NCT01641939).
Everolimus is an oral mTOR inhibitor that demonstrated promising efficacy in a phase II study of pretreated aGC. An international double-blind phase III study compared Everolimus efficacy and safety with best supportive care (BSC) in previously treated aGC. Everolimus did not significantly improve OS after one or two lines of previous systemic chemotherapy. Its efficacy seems to be limited only to a subset of patients harboring PI3K and pS6 aberrations, potentially identifying these lesions as possible biomarkers (76,77).
Consideration and future perspectives
Despite the global downward trends in GC mortality, this malignancy is still one of the most highly prevalent leading causes of cancer-related death worldwide. This sad supremacy is due to multiple conditions, mostly related with the late time of the diagnosis, the paucity of premonitory symptoms and the high variability of genomic changes occurring in GC cells, from the first tumorigenic steps to the final acquisition of malignant and metastatic phenotype. For all these reasons, the treatment options are limited, and there is the urgent need to identify novel and specific markers for diagnosis, predictors of GC survival and overall new therapeutic strategies for treatment.
High-throughput approaches of exome and transcriptome wide analyses have identified new target genes potentially and, more importantly, consolidated the supremacy of known molecules already object of targeted therapies, identifying the key pathways commonly used by cancer cells to sustain oncogenic programs. The EGFR family provides oncogenic signals to epithelial cells by virtue of structural mutation, hyper-expression and gene amplification, facts that justify the continue effort to develop drugs, inhibitors and mAbs against these receptors, particularly ErbB2 and its downstream transducers. However, development of resistance to anti-ErbB2 mono-therapies remains the major hurdle to overcome.
In principle, two can be the major mechanisms of resistance to anti ErbB2 therapy: an ex novo resistance, due to the occurrence of genetic alterations during tumor progression, occurring also in the ErbB2 signaling pathway; and acquired resistance, primarily due to the activation of alternative pathways to compensate for ErbB2 inhibition.
The network organization of the ErbB1-4 receptors appears to be ideally organized to supply mechanisms of escape from targeted-mono-therapies, due to their ability to aggregate in different dimer combination. This peculiar attitude confers robustness and redundancy to the signaling throughput, which is kept in shape even in relatively paucity of either one receptor.
In cancer cells the administration of trastuzumab while disrupting the ErbB2 dimers, favors its aggregation with ErbB3. Heterodimers of ErbB2 and ErbB3 have been suggested to contain the highest growth and transforming potential of any dimer combination for the ability to recruit the largest set of agonistic signaling molecules, as compared to any other dimers containing ErbB2. This is more than hypothesis, and is actually one of the driving rationales for the “dual hit strategy” to combine pertuzumab and trastuzumab to efficiently target, no more the ErbB2 receptor, but the dimers containing-ErbB2.
These observations highlight at least two other aspects that need to be considered: (I) the expression profile of the ErbB receptors, which is different for any given tumor; (II) the opportunity to target ErbB3, the preferred partner of ErbB2.
To the first aim, the molecular profiling of tumor cells to determine the relative levels of EGFR family members and their ligands, might be useful to predict sensitivity to trastuzumab or pertuzumab, and eventually decide which one to use first, if not the combination of the two. In fact, the sharp peculiar differences in the binding ability of these two mAbs to ErbB2 makes them precious tools that can be used in combination to control dimer formation.
The ErbB3 receptor is activated in allosteric conformation by its kinase active partner receptors, and works as a dynamic signaling hub linking their signals to the downstream PI3K/Akt signaling pathway. As such, the expression of ErbB3 is a potential point of impact in tumorigenic signaling and in drug resistance. These predictions have been validated in several experimental models. Although the proof of concept is not been achieved yet, the expectation arising from workbench and preclinical studies, for clinical relevant use of mAbs against ErbB3 in cancer therapy, alone or most likely in combination with anti-ErbB2 drugs, is high.
In principle, the best strategy to target a receptor devoid of intrinsic kinase activity like ErbB3 is to generate mAbs, and probably the best successful mAb would be able to promote receptor internalization and degradation (78,79). Its functions would have impact on signal attenuation of both PI3K and ERK pathways, and favor the ErbB2 redistribution towards homodimers formation, leading to the possibility to target them with trastuzumab. However, a low percentage of tumors express mutations in the ErbB3 ECD able to activate its kinase in absence of heterodimerization, and for these, kinase inhibitors will be better drugs.
In conclusion, although trastuzumab has been approved for treatment of ErbB2-positive GC, a significant proportion of patients did not respond or developed acquired resistance to this mAb (ToGA trial), indicating that an ErbB2 targeted mono-therapy can be elusive, leading to the activation of compensatory pathways in GC cells. Therefore, understanding mechanisms of resistance to ErbB2-target therapy is expected to identify criteria for choosing treatments, more rational and less empiric. Due to the complex regulation of the ErbB receptors composition occurring during prolonged targeted therapies, monitoring of the ErbB-related genetic feedbacks regulating receptor level expression and pathway activation may be strategic to control efficacy and toxicity of anticancer agents. Furthermore, the availability of therapeutic mAbs anti ErbB3 to synergistically block multiple ErbB receptor dimers will speed up knowledge on this field, opening a new frontier for the ErbB-targeted therapy of cancer.
Acknowledgements
Dr. P Malaguti MD, Ph.D. is supported by the Istituto Regina Elena Cancer Center, Oncologia Medica A, of Rome. Dr. MM D’Aloia is PhD student at the Department of Clinical and Molecular Medicine at the “Sapienza University of Rome”. Dr. M Alimandi is Professor at the “Sapienza University of Rome” and is supported by AIRC RG 1133.
Footnote
Conflicts of Interest: The authors have no conflicts of interest to declare.
References
- Jemal A, Center MM, DeSantis C, et al. Global patterns of cancer incidence and mortality rates and trends. Cancer Epidemiol Biomarkers Prev 2010;19:1893-907. [PubMed]
- Siegel R, Naishadham D, Jemal A. Cancer statistics, 2013. CA Cancer J Clin 2013;63:11-30. [PubMed]
- Guilford P, Hopkins J, Harraway J, et al. E-cadherin germline mutations in familial gastric cancer. Nature 1998;392:402-5. [PubMed]
- Caldas C, Carneiro F, Lynch HT, et al. Familial gastric cancer: overview and guidelines for management. J Med Genet 1999;36:873-80. [PubMed]
- Carneiro F, Charlton A, Huntsman DG. Hereditary diffuse gastric cancer. In: Bosman FT, Carneiro F, Hruban RH, et al. WHO classification of tumours of the digestive system. Fourth edition. Lyon: IARC, 2010:59-63.
- Worthley DL, Phillips KD, Wayte N, et al. Gastric adenocarcinoma and proximal polyposis of the stomach (GAPPS): a new autosomal dominant syndrome. Gut 2012;61:774-9. [PubMed]
- Lauwers GY, Carneiro F, Graham DY, et al. Gastric carcinoma. In: Bosman FT, Carneiro F, Hruban RH, et al. WHO classification of tumours of the digestive system. Fourth edition. Lyon: IARC, 2010:48-58.
- Malfertheiner P, Peitz U. The interplay between Helicobacter pylori, gastro-oesophageal reflux disease, and intestinal metaplasia. Gut 2005;54:i13-20. [PubMed]
- Lei Z, Tan IB, Das K, et al. Identification of molecular subtypes of gastric cancer with different responses to PI3-kinase inhibitors and 5-fluorouracil. Gastroenterology 2013;145:554-65. [PubMed]
- Yang W, Raufi A, Klempner SJ. Targeted therapy for gastric cancer: molecular pathways and ongoing investigations. Biochim Biophys Acta 2014;1846:232-7.
- Jácome AA, Wohnrath DR, Scapulatempo Neto C, et al. Prognostic value of epidermal growth factor receptors in gastric cancer: a survival analysis by Weibull model incorporating long-term survivors. Gastric Cancer 2014;17:76-86. [PubMed]
- Deng N, Goh LK, Wang H, et al. A comprehensive survey of genomic alterations in gastric cancer reveals systematic patterns of molecular exclusivity and co-occurrence among distinct therapeutic targets. Gut 2012;61:673-84. [PubMed]
- Murase H, Inokuchi M, Takagi Y, et al. Prognostic significance of the co-overexpression of fibroblast growth factor receptors 1, 2 and 4 in gastric cancer. Mol Clin Oncol 2014;2:509-17. [PubMed]
- Hayashi M, Inokuchi M, Takagi Y, et al. High expression of HER3 is associated with a decreased survival in gastric cancer. Clin Cancer Res 2008;14:7843-9. [PubMed]
- Wu X, Chen Y, Li G, et al. Her3 is associated with poor survival of gastric adenocarcinoma: Her3 promotes proliferation, survival and migration of human gastric cancer mediated by PI3K/AKT signaling pathway. Med Oncol 2014;31:903. [PubMed]
- Tang D, Liu CY, Shen D, et al. Assessment and prognostic analysis of EGFR, HER2, and HER3 protein expression in surgically resected gastric adenocarcinomas. Onco Targets Ther 2014;8:7-14. [PubMed]
- Wang YK, Gao CF, Yun T, et al. Assessment of ERBB2 and EGFR gene amplification and protein expression in gastric carcinoma by immunohistochemistry and fluorescence in situ hybridization. Mol Cytogenet 2011;4:14. [PubMed]
- Bang YJ, Van Cutsem E, Feyereislova A, et al. Trastuzumab in combination with chemotherapy versus chemotherapy alone for treatment of HER2-positive advanced gastric or gastro-oesophageal junction cancer (ToGA): a phase 3, open-label, randomised controlled trial. Lancet 2010;376:687-97. [PubMed]
- Citri A, Yarden Y. EGF-ERBB signalling: towards the systems level. Nat Rev Mol Cell Biol 2006;7:505-16. [PubMed]
- Alimandi M, Romano A, Curia MC, et al. Cooperative signaling by ErbB3 and ErbB2 in neoplastic transformation and human malignancies. Oncogene 1995;10:1813-21. [PubMed]
- Wong H, Yau T. Targeted therapy in the management of advanced gastric cancer: are we making progress in the era of personalized medicine? Oncologist 2012;17:346-58. [PubMed]
- Stein RA, Staros JV. Insights into the evolution of the ErbB receptor family and their ligands from sequence analysis. BMC Evol Biol 2006;6:79. [PubMed]
- Shelly M, Pinkas-Kramarski R, Guarino BC, et al. Epiregulin is a potent pan-ErbB ligand that preferentially activates heterodimeric receptor complex. J Biol Chem 1998;273:10496-505. [PubMed]
- Alimandi M, Wang LM. Epidermal growth factor and betacellulin mediate signal transduction trough co-expressed ErbB-2 and ErbB-3 receptors. EMBO J 1997;16:5608-17. [PubMed]
- Pinkas-Kramarski R, Shelly M, Guarino BC, et al. ErbB tyrosyne kinase and the two neuregulin families constitute a ligand-receptor network. Mol Cell Biol 1998;18:6090-101. [PubMed]
- Hijazi MM, Young PE, Dougherty MK, et al. NRG-3 in human breast cancers: Activation of multiple ErbB family proteins. Int J Oncol 1998;13:1061-7. [PubMed]
- Landgraf R. HER2 therapy. HER2 (ERBB2): functional diversity from structurally conserved building blocks. Breast Cancer Res 2007;9:202. [PubMed]
- Flågeng MH, Knappskog S, Haynes BP, et al. Inverse regulation of EGFR/HER1 and HER2-4 in normal and malignant human breast tissue. PLoS One 2013;8:e74618. [PubMed]
- Baselga J, Swain SM. Novel anticancer targets: revisiting ERBB2 and discovering ERBB3. Nat Rev Cancer 2009;9:463-75. [PubMed]
- Osaki M, Oshimura M, Ito H. PI3K-Akt pathway: its functions and alterations in human cancer. Apoptosis 2004;9:667-76. [PubMed]
- Zhang XL, Yang YS, Xu DP, et al. Comparative study on overexpression of HER2/neu and HER3 in gastric cancer. World J Surg 2009;33:2112-8. [PubMed]
- Iorio MV, Casalini P, Piovan C, et al. microRNA-205 Regulates HER3 in human breast cancer. Cancer Res 2009;69:2195-200. [PubMed]
- Scott GK, Goga A, Bhaumik D, et al. Coordinate suppression of ERBB2 and ERBB3 by enforced expression of micro-RNA miR-125a or miR-125b. J Biol Chem 2007;282:1479-86. [PubMed]
- Garrett JT, Olivares MG, Rinehart C, et al. Transcriptional and posttranslational up-regulation of HER3 (ErbB3) compensates for inhibition of the HER2 tyrosine kinase. Proc Natl Acad Sci USA 2011;108:5021-6. [PubMed]
- Chandarlapaty S, Sawai A, Scaltriti M, et al. AKT Inhibition Relieves Feedback Suppression of Receptor Tyrosine Kinase Expression and Activity. Cancer Cell 2011;19:58-71. [PubMed]
- Stephens PJ, Tarpey PS, Davies H, et al. Oslo Breast Cancer Consortium (OSBREAC). The landscape of cancer genes and mutational processes in breast cancer. Nature 2012;486:400-4. [PubMed]
- Jaiswal BS, Kljavin NM, Stawiski EW, et al. Oncogenic ERBB3 mutations in human cancers. Cancer Cell 2013;23:603-17. [PubMed]
- Wilson TR, Fridlyand J, Yan Y, et al. Widespread potential for growth-factor-driven resistance to anticancer kinase inhibitors. Nature 2012;487:505-9. [PubMed]
- Engelman JA, Zejnullahu K, Mitsudomi T, et al. MET amplification leads to gefitinib resistance in lung cancer by activating ERBB3 signaling. Science 2007;316:1039-43. [PubMed]
- Abdel-Rahman O. Targeting the hepatocyte growth factor/mesenchymal epithelial transition pathway in gastric cancer: biological rationale and clinical applications. Expert Rev Anticancer Ther 2015;15:235-45. [PubMed]
- Fioroni I, Dell’Aquila E, Pantano F, et al. Role of c-mesenchymal-epithelial transition pathway in gastric cancer. Expert Opin Pharmacother 2015;16:1195-207. [PubMed]
- Kuniyasu H, Yasui W, Kitadai Y, et al. Frequent amplification of the c-met gene in scirrhous type stomach cancer. Biochem Biophys Res Commun 1992;189:227-32. [PubMed]
- Nakajima M, Sawada H, Yamada Y, et al. The prognostic significance of amplification and overexpression of c-met and c-erbB-2 in human gastric carcinomas. Cancer 1999;85:1894-902. [PubMed]
- Lee JH, Han SU, Cho H, et al. A novel germ line juxtamembrane Met mutation in human gastric cancer. Oncogene 2000;19:4947-53. [PubMed]
- Janbabai G, Oladi Z, Farazmandfar T, et al. The prognostic impact of EGFR, ErbB2 and MET gene amplification in human gastric carcinomas as measured by quantitative Real-Time PCR. J Cancer Res Clin Oncol 2015. [Epub ahead of print]. [PubMed]
- Hao NB, Tang B, Wang GZ, et al. Hepatocyte growth factor (HGF) upregulates heparanase expression via the PI3K/Akt/NF-κB signaling pathway for gastric cancer metastasis. Cancer Lett 2015;361:57-66. [PubMed]
- Guo A, Villén J, Kornhauser J, et al. Signaling networks assembled by oncogenic EGFR and c-Met. Proc Natl Acad Sci USA 2008;105:692-7. [PubMed]
- Xu Y, Liu H, Chen J, et al. Acquired resistance of lung adenocarcinoma to EGFR-tyrosine kinase inhibitors gefitinib and erlotinib. Cancer Biol Ther 2010;9:572-82. [PubMed]
- Bean J, Brennan C, Shih JY, et al. MET amplification occurs with or without T790M mutations in EGFR mutant lung tumors with acquired resistance to gefitinib or erlotinib. Proc Natl Acad Sci USA 2007;104:20932-7. [PubMed]
- Corso S, Ghiso E, Cepero V, et al. Activation of HER family members in gastric carcinoma cells mediates resistance to MET inhibition. Mol Cancer 2010;9:121. [PubMed]
- Fuse N, Kuboki Y, Kuwata T, et al. Prognostic impact of HER2, EGFR, and c-MET status on overall survival of advanced gastric cancer patients. Gastric Cancer 2015. [Epub ahead of print]. [PubMed]
- Shi J, Yao D, Liu W, et al. Highly frequent PIK3CA amplification is associated with poor prognosis in gastric cancer. BMC Cancer 2012;12:50. [PubMed]
- Lee J, van Hummelen P, Go C, et al. High-throughput mutation profiling identifies frequent somatic mutations in advanced gastric adenocarcinoma. PLoS One 2012;7:e38892. [PubMed]
- Sukawa Y, Yamamoto H, Nosho K, et al. HER2 expression and PI3K-Akt pathway alterations in gastric cancer. Digestion 2014;89:12-7. [PubMed]
- Zaitsu Y, Oki E, Ando K, et al. Loss of heterozygosity of PTEN (encoding phosphate and tensin homolog) associated with elevated HER2 expression is an adverse prognostic indicator in gastric cancer. Oncology 2015;88:189-94. [PubMed]
- Zhang X, Park JS, Park KH, et al. PTEN deficiency as a predictive biomarker of resistance to HER2-targeted therapy in advanced gastric cancer. Oncology 2015;88:76-85. [PubMed]
- Cho HS, Mason K, Ramyar KX, et al. Structure of the extracellular region of her2 alone and in complex with the hercepti fab. Nature 2003;421:756-60. [PubMed]
- Junttila TT, Akita RW, Parsons K, et al. Ligand-independent HER2/HER3/PI3K complex is disrupted by Trastuzumab and is effectively inhibited by the PI3K inhibitor GDC-0941. Cancer Cell 2009;15:429-40. [PubMed]
- Franklin MC, Carey KD, Vajdos FF, et al. Insights into erbb signaling from the structure of the erbb2-Pertuzumab complex. Cancer Cell 2004;5:317-28. [PubMed]
- Agus DB, Akita RW, Fox WD, et al. Targeting ligand-activated ErbB2 signaling inhibits breast and prostate tumor growth. Cancer Cell 2002;2:127-37. [PubMed]
- Ghosh R, Narasanna A, Wang SE, et al. Trastuzumab has preferential activity against breast cancers driven by HER2 homodimers. Cancer Res 2011;71:1871-82. [PubMed]
- Austin CD, De Mazière AM, Pisacane PI, et al. Endocytosis and sorting of ErbB2 and the site of action of cancer therapeutics Trastuzumab and geldanamycin. Mol Biol Cell 2004;15:5268-82. [PubMed]
- Goltsov A, Deeni Y, Khalil HS, et al. Systems analysis of drug-induced receptor tyrosine kinase reprogramming following targeted mono- and combination anti-cancer therapy. Cells 2014;3:563-91. [PubMed]
- Sergina NV, Rausch M, Wang D, et al. Escape from HER-family tyrosine kinase inhibitor therapy by the kinase-inactive HER3. Nature 2007;445:437-41. [PubMed]
- Nahta R, Hung MC, Esteva FJ. The HER-2-targeting antibodies Trastuzumab and Pertuzumab synergistically inhibit the survival of breast cancer cells. Cancer Res 2004;64:2343-6. [PubMed]
- Scheuer W, Friess T, Burtscher H, et al. Strongly enhanced antitumor activity of Trastuzumab and Pertuzumab combination treatment on HER2-positive human xenograft tumor models. Cancer Res 2009;69:9330-6. [PubMed]
- Yamashita-Kashima Y, Iijima S, Yorozu K, et al. Pertuzumab in combination with Trastuzumab shows significantly enhanced antitumor activity in HER2-positive human gastric cancer xenograft models. Clin Cancer Res 2011;17:5060-70. [PubMed]
- Sims AH, Zweemer AJ, Nagumo Y, et al. Defining the molecular response to Trastuzumab, Pertuzumab and combination therapy in ovarian cancer. Br J Cancer 2012;106:1779-89. [PubMed]
- Swain SM, Kim SB, Cortés J, et al. Pertuzumab, Trastuzumab, and docetaxel for HER2-positive metastatic breast cancer (CLEOPATRA study): overall survival results from a randomised, double-blind, placebo-controlled, phase 3 study. Lancet Oncol 2013;14:461-71. [PubMed]
- Kang YK, Rha SY, Tassone P, et al. A phase IIa dose-finding and safety study of first-line Pertuzumab in combination with Trastuzumab, capecitabine and cisplatin in patients with HER2-positive advanced gastric cancer. Br J Cancer 2014;111:660-6. [PubMed]
- Lorenzen S, Riera Knorrenschild J, Haag GM, et al. Lapatinib versus Lapatinib plus capecitabine as second-line treatment in human epidermal growth factor receptor 2-amplified metastatic gastro-oesophageal cancer: a randomised phase II trial of the Arbeitsgemeinschaft Internistische Onkologie. Eur J Cancer 2015;51:569-76. [PubMed]
- Bang YJ, Yalcin S, Roth A, et al. Registry of gastric cancer treatment evaluation (REGATE): I baseline disease characteristics. Asia Pac J Clin Oncol 2014;10:38-52. [PubMed]
- Shah MA, Wainberg ZA, Catenacci DV, et al. Phase II study evaluating 2 dosing schedules of oral foretinib (GSK1363089), cMET/VEGFR2 inhibitor, in patients with metastatic gastric cancer. PLoS One 2013;8:e54014. [PubMed]
- Barok M, Tanner M, K[UNKNOWN ENTITY &odie;]ninki K, et al. Trastuzumab-DM1 is highly effective in preclinical models of HER2-positive gastric cancer. Cancer Lett 2011;306:171-9. [PubMed]
- Burris HA 3rd, Tibbitts J, Holden SN, et al. Trastuzumab emtansine (T-DM1): a novel agent for targeting HER2+ breast cancer. Clin Breast Cancer 2011;11:275-82. [PubMed]
- Park JH, Ryu MH, Park YS, et al. Successful control of heavily pretreated metastatic gastric cancer with the mTOR inhibitor everolimus (RAD001) in a patient with PIK3CA mutation and pS6 overexpression. BMC Cancer 2015;15:119. [PubMed]
- Ohtsu A, Ajani JA, Bai YX, et al. Everolimus for previously treated advanced gastric cancer: results of the randomized, double-blind, phase III GRANITE-1 study. J Clin Oncol 2013;31:3935-43. [PubMed]
- Gaborit N, Abdul-Hai A, Mancini M, et al. Examination of HER3 targeting in cancer using monoclonal antibodies. Proc Natl Acad Sci USA 2015;112:839-44. [PubMed]
- Aurisicchio L, Marra E, Luberto L, et al. Novel anti-ErbB3 monoclonal antibodies show therapeutic efficacy in xenografted and spontaneous mouse tumors. J Cell Physiol 2012;227:3381-8. [PubMed]