KRAS, BRAF and gastric cancer
Introduction
Gastric cancer (GC) is a common cancer with a worldwide incidence of nearly one million cases per year (1). In 2012, there were an estimated 723,100 GC deaths worldwide, making GC the third most frequent cause of cancer related death. There is a large geographic variation in GC incidence, with the highest incidence rates in Eastern Asia (particularly in Korea, Mongolia, Japan, and China), Central and Eastern Europe, and South America and lowest rates in Northern America and most parts of Africa. The incidence of GC in men is about twice as high as in women (2) and approximately 10% of GCs have a familial component (3). Helicobacter pylori (H. pylori) infection is an established risk factor for developing GC. 89% of cases of non-cardia GC worldwide are attributed to this bacterium (4). Survival of GC patients remains poor. The overall 5-year survival of patients with locally advanced unresectable, recurrent or metastatic GC is 5-20% if treated with cytotoxic chemotherapy (5), increasing to 36% in patients with locally advanced resectable GC treated with peri-operative chemotherapy followed by surgery (6). Thus, there is an urgent clinical need to identify new potential drug targets in order to improve survival for GC patients.
Macroscopically, GCs are categorized according to the Borrmann classification into type I (polypoid), type II (fungating), type III (ulcerating), and type IV (diffusely infiltrating) (7). Histologically, GCs are most commonly categorized using the Lauren classification into intestinal, diffuse and mixed/indeterminate type (8). The intestinal-type occurs more commonly in elderly patients, whereas the diffuse-type is seen in particular in young female patients and has a poorer prognosis (9). In the West, the relative proportion of intestinal-type GC is up to 74% intestinal-type (10) compared to 44% in the East (11). Staging of GC is performed using the International Union Against Cancer (UICC) (12), American Joint Committee on Cancer (AJCC) (13) or Japanese Gastric Cancer Association (JGCA) (14) Tumor Node Metastasis (TNM) staging system which follow same principals but have some minor variations.
Molecular aberrations are known to play an important role in the development of GC. In addition to mutations in oncogenes, such as TP53, APC, CDH1, p16 and PTEN, or tumor suppressor genes such as β-catenin, BRAF, KRAS, PIK3CA and ERBB2 (15), microsatellite instability (MSI) caused by deficient DNA mismatch repair (MMR) has been identified in 15% to 30% GC (16). DNA aneuploidy, a surrogate marker for chromosomal instability, has been reported in 24-85% GC (17) and Epstein-Barr Virus (EBV) infection has been identified in approximately 9% GCs (18). Several different molecular classifications of GCs have been proposed recently (19). For a recent review on this subject see Tan et al. (20).
The focus of this review is on the existing literature on genetic alterations in KRAS and BRAF in GC. Reported incidence of mutations in KRAS and BRAF and their relation to clinicopathological and molecular variables including KRAS amplification are analyzed and summarized. Literature on KRAS/BRAF epigenetic changes has been excluded from this review. Results from GC are compared with studies investigating KRAS and BRAF mutations in CRC and cancer of the small bowel. Furthermore, the clinical relevance of determining the mutational status and DNA copy number of these genes in relation to GC patient treatment will be discussed.
Methods
The Web of Science (from 1988-14th May 2015) and PubMed (from 1946-14th May 2015) databases were searched for all known gene aliases of KRAS and BRAF (gene aliases from www.genecards.org, accessed on 8th May 2015). These aliases were used as search terms in combination with (‘‘gastric cancer’’ or ‘‘stomach cancer’’ or ‘‘gastric carcinoma’’ or ‘‘stomach carcinoma’’, see Table 1).
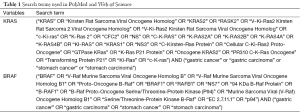
Full table
Eligibility to be included in the current review was restricted to original articles reporting GC studies using human tissue, blood or plasma samples irrespective of sample size and stage of disease. Other tumors of the stomach such as lymphomas or gastrointestinal (GI) stromal tumors, and cell line studies were excluded. The reference lists of publications included in this review were searched for further relevant articles. Each article was analyzed for information on study size, geographical origin of patient cohort (East versus West), age, gender, survival, and whether any chemo(radio)therapy was given. With regard to DNA isolation from tumor tissue, the reported tumor cell density, number of blocks used, and tissue processing [frozen versus formalin-fixed paraffin embedded (FFPE)] were analyzed. Furthermore, information on the mutation incidence, the mutation detection method and investigated codons was collected from each study. The relationship of mutation status with clinicopathological variables, DNA MMR status and MSI, and DNA ploidy was noted.
Results
The initial database searches found 1,369 articles in total. After screening, applying exclusion criteria and including additional articles from references, the final number of articles used for this review was 69. For a Preferred Reporting Items for Systematic Reviews and Meta-Analyses (PRISMA) flow diagram illustrating the manuscript selection process, see Figure 1.
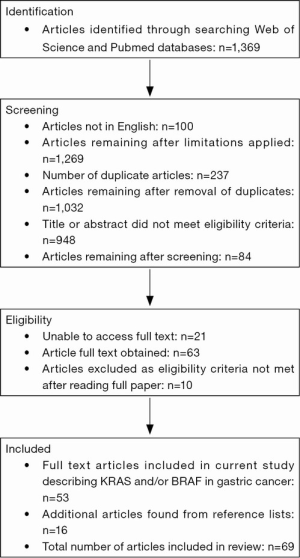
The KRAS
Mammalian cells encode three functional RAS genes: HRAS, KRAS and NRAS (21,22). Although these different isoforms share a similar structure, their expression and/or activation differs by tissue and cancer types (23-25). This review will focus on KRAS as it is the most frequently mutated RAS gene in GC (26).
Kirsten Rat Sarcoma Viral Oncogene Homolog (KRAS) was discovered in 1982 by Chang et al. (21). KRAS is a tumor suppressor gene which is located on chromosome 12p12 (www.genecards.org, accessed 8th May 2015). It has six exons and alternative splicing of exon 4 produces KRAS4A and KRAS4B which contains 188 and 189 amino acids, respectively (27). KRAS encodes a small guanosine triphosphatase (GTPase) protein with a molecular mass of 21.6 kD (28).
The KRAS protein contains four domains which determine the interaction with GTP (G-domain, amino acids 1-165), the anchoring of the protein in the plasma membrane (hypervariable region at the C-terminus, amino acids 165-188) as well as the binding of other regulators and effectors such as RAF and PI3K (28).
KRAS cycles between an inactive GDP-bound state and an active GTP-bound state (29). Activation of KRAS is triggered through a number of different types of receptors including tyrosine kinase receptors such as epidermal growth factor receptor (EGFR), as well as cytokine receptors, T cell receptors, and subunits of heterotrimeric G proteins (30). Active RAS-GTP undergoes a conformational change affecting its interaction with various downstream effector molecules such as RAF and mitogen-activated protein kinase (MAPK) (31) or PI3K/AKT (32). This in turn activates nuclear transcription factors inducing a cascade of cellular processes such as proliferation, angiogenesis, apoptosis, or cell survival (26). Mutant KRAS functions as an oncogene inducing malignant transformation of cells due to permanent activation of downstream effectors (33).
KRAS mutations have been found in many human cancers. The most common mutations are located in codon 12 or 13 in exon 1, and less frequently in codon 61, 63, 117, 119 and 146 (28). Mutations in codons 12 and 13 are known to result in conformational changes and permanent expression (‘activation’) of the KRAS protein (34). Overexpression of KRAS as a result of loss of p16INK4 or loss of p53 has also been reported (35). For a more general review on KRAS mutations in human cancer, see Jancik et al. (28).
KRAS in GC
KRAS mutations
The first report of a KRAS mutation in a single GC was published in 1986. Investigators described the presence of a single mutated KRAS allele (gly-12 to ser), together with a 30-50 fold amplification of the other KRAS allele (36).
Since this first publication, 64 studies have reported on the incidence of KRAS mutations in GC, with the majority of studies (61%) originating from Asia (see Tables 2,3). Two studies compared KRAS mutations between GC patients from the East and the West (37,38). Forty-five (70%) studies investigated the KRAS mutation status in patient cohorts comprising less than 100 patients.
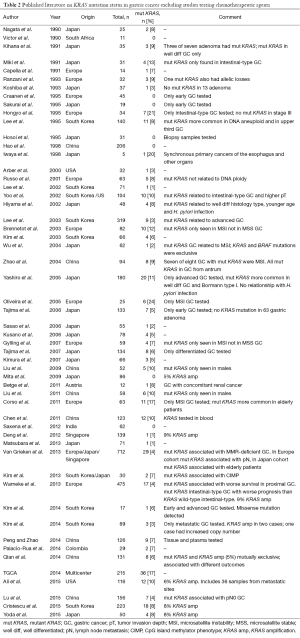
Full table
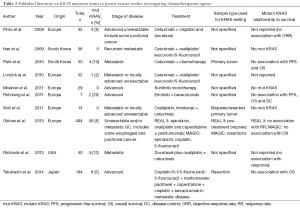
Full table
GC cohorts
The median number of patients per study was 61, ranging from 5 to 712 patients. Excluding three international multicenter studies and two studies that did not mention the geographical origin of their patients, there were 39 (66%) studies from the East and 22 (37%) studies from the West. Studies from the East had a higher median study size of 66 patients, ranging from 5 to 319 patients compared to studies from the West with a median study size of 33 patients, ranging from 7 to 494 patients. The largest GC study was an international multicenter study including 712 GCs: 278 GC from the United Kingdom, 230 GC from Japan and 204 CG from Singapore (38).
Twenty-five (39%) studies performed KRAS testing on samples from multiple centers (19,37-60), 20 (31%) studies used samples from a single center (61-80), and the remaining did not report this information. Twenty-seven (42%) studies were performed using DNA extracted from formalin-fixed paraffin (37-39,41,42,44,45,47,48,50-52) embedded tissue samples (56,61,63-66,68,69,72-74,81-84). With the exception of 11 studies which did not report at all which tissue was used (40,54,77,80,85-91), all other studies used DNA from ‘paraffin embedded tissue’ (fixation method not reported) (43,92-94), frozen tissue (19,46,53,59,60,67,70,71,75,76,78,79,95-98), blood or plasma samples (99), or a combination of the above (49,55,57,58,62). Of the studies using tissue samples, 37 (59%) used DNA extracted (38,39,44,46,47,50,52-54,60-64,67,68) from resection specimens (70,71,73-76,78-82,84,88-91,93,95-98), ten (16%) used a combination of biopsy and resection specimens (37,40,45,51,65,69,72,87,92,94) and two (3%) used biopsy specimens (77,86). The remaining 14 (22%) did not report on the type of specimen used (19,41-43,48,49,55-59,66,83,85). No study reported extracting DNA from multiple blocks, thus we have assumed that all studies used a single block for DNA extraction. Thirty-seven (59%) studies considered the tumor cell density of the tissue prior to DNA extraction by either performing microdissection or preselecting areas of tumor with tumor cell density ranging from >20% to >80% (19,37-40,44,46-54,61,62,64-71,73-76,81,82,84,89,93,94,98). Twenty-two (34%) studies investigated only subgroups of GC patients, thus eight (36%) studies investigated locally advanced GC (40-44,61,62,82), four (18%) studies metastatic and advanced GC (48,49,81,94), three (14%) studies early GC (45,65,84), two (9%) studies metastatic GC (66,90), two (9%) studies compared early with advanced GC (46,93), one (5%) study intestinal GC (47), one (5%) study MSI GC (85) and one study (5%) investigated GC with concomitant renal cancer (63).
KRAS mutation detection methods
A wide variety of methods was used to detect KRAS mutations. Twenty-six (41%) studies used polymerase chain reaction (PCR) (37,43,44,49,60,61,66,70,74,75,80,88,98) or single-strand conformation polymorphism (SSCP) (39,45,47,52,64,65,71,72,85,93,95,97,99) for mutation screening, followed by confirmatory direct Sanger sequencing. Other methods used to detect KRAS mutations included restriction fragment length polymorphism (RFLP) (51,76-78,83,86), next-generation sequencing (NGS) (19,46,48,59,67,81,87,96), pyrosequencing (63,68), Q-PCR (41,94), nested and COLD-PCR (55), denaturing gradient gel electrophoresis (DGGE) (89,91), dot blot hybridization assay (56-58,69,73,82), high-resolution melting analysis (HRMA) (42,50,53,54) and direct Sanger sequencing (62,79). The largest international multicenter study used a combination of HRMA followed by Sanger sequencing, pyrosequencing, and MassARRAY (38). One study used RFLP and SSCP followed by direct sequencing (92), while other studies used a combination of RFLP and dot blot hybridization (84) or a combination of Q-PCR and Sanger sequencing (40). One study did not report which KRAS mutation detection method was used (90).
Investigated KRAS codons
Excluding eight studies that performed whole genome sequencing, 49 (88%) studies published information on investigated codons for mutation testing. The remaining seven (13%) studies did not provide any information which codons they investigated, however, they later report only mutations in specific codons. All studies investigated multiple codons, with 49 (100%) investigating codon 12, 45 (92%) codon 13, 18 (37%) codon 61, and 1 codon 146. Only a single study investigated all four codons (codons 12, 13, 61 and 146) (62) and one study investigated codon 59, in addition to codons 12, 13 and 61 (93).
Incidence of KRAS mutations
The overall median incidence of a KRAS mutation in GC was 6.5% ranging from 0-29%. The median KRAS incidence was similar in studies from the East and the West (East: 6%, ranging from 0-20%; West 7.5%, ranging from 0-29%). Likewise, the largest international multicenter study reported an overall incidence of KRAS mutations of 4.2% which did not differ between Eastern and Western countries (UK: 6%, Japan 4%, Singapore 2%) (38).
Of the 36 studies that reported the location of the mutations in KRAS, 154 mutations were found in codon 12, 66 mutations in codon 13, six mutations in codon 61. No mutation has been found so far in codon 146. The only study to report KRAS mutations in codon 11, was the result of SSCP and direct sequencing of exon 1. This revealed that two of the seven mutations found in 34 GCs were located in codon 11, all other mutations were in codons 12 and 13 (47). Another study, in addition to identifying one KRAS mutation in codon 12 and two KRAS mutations in codon 13, also found a K5N mutation in exon 2 and five A59T mutations in exon 4 (93). There was only a single report of a single GC having multiple mutations in codon 12 and codon 13 (78).
KRAS mutation status and clinicopathological variables
Twenty-nine (45%) studies have investigated the relationship between KRAS mutation status (19,37,38,40,46,47,50-54,56,60,62-64) and one or more clinicopathological variables (68,69,71-73,75,76,82,88,91,93,96,98). These included grade of tumor differentiation, Lauren classification, tumor location, tumor invasion depth (pT), lymph node status (pN), Borrmann classification, age, gender, and infection with H. pylori or EBV. The most frequent investigated association was found between KRAS mutation status and pT, followed by gender and age reported in 33%, 30% and 30% of studies, respectively.
KRAS mutation and age
Nineteen (30%) studies investigated the relationship between patient age and KRAS mutation status mostly suggesting that KRAS mutations are more frequent in elderly GC patients. Seven (37%) studies reported individual ages or the median age of patients with a KRAS mutation (19,46,60,62,63,69,96), whereas the remaining studies stratified patient age into a range of subcategories (38,50,52-55,68,72,76). Only Hiyama et al. reported a significantly higher incidence of KRAS mutations in patients younger than 60 years (72). One study reported an equal number of KRAS mutations in patients ≤65 years old and >65 years old (54). All other studies found KRAS mutations more frequently in elderly patients although this association often did not reach statistical significance (38,50,52,53,55,68,76,98).
KRAS mutation and gender
Nineteen (30%) studies investigated the relationship between gender and KRAS mutation status in GC. Although no statistically significant relationship between KRAS mutation status and gender was found, most studies seem to suggest that KRAS mutations are more frequent in males. Nine (47%) studies found a higher incidence in males (38,46,50,55,62,68,69,72,76), three (16%) studies reported that KRAS mutations were exclusively found in males (53,54,63) whereas four (21%) studies found an equal incidence of KRAS mutations in males and females (60,75,91,96).
KRAS mutation and tumor location
Twelve (19%) studies investigated the relationship between KRAS mutation status and GC location within the stomach. Tumors in the upper third of the stomach had a significantly higher incidence of KRAS codon 12 mutations compared to GCs in the middle or lower (3%) third of the stomach (76). Summarizing and interpreting the results from the other studies is difficult as stomach area categorization varied substantially between studies. We therefore defined that GCs located in the cardia or upper third are ‘proximal’ and GCs located in all other regions are ‘distal’. These studies found a higher incidence of KRAS mutations in distal GC (19,37,38,60,63,64,68,72,75,91).
KRAS mutation and Borrmann classification
A single study investigated the relationship between KRAS mutation status and macroscopic classification according to Borrmann. This study investigated KRAS codons 12 and 13 in 108 GC patients with advanced disease and found a significant relationship between KRAS mutation status and Borrmann Type 1 (polypoid) GC (82). The incidence of KRAS mutation was 6/14 (43%), 8/29 (28%), 2/11 (18%), and 4/54 (7%) in Borrmann type 1 to 4 GCs, respectively. Interestingly all KRAS mutations in polypoid GCs were located in codon 12. This is in contrast to a study investigating 48 GC which did not find any relationship between macroscopic appearance (classified according to the Japanese Research Society for Gastric Cancer) and KRAS mutation status (72).
KRAS mutation and primary tumor invasion depth (pT category)
Twenty-one (33%) studies investigated the relationship between KRAS mutation status and pT in GC. Unfortunately, different staging systems were used in different publications and some studies compared groups of pT categories against each other making the results interpretation difficult. None of the studies reported a significant association between pT category/stage and KRAS mutation status. Overall, there was a higher incidence of KRAS mutations in higher pT (pT2-4) GC compared to lower pT (pT1) GC (19,37,38,47,50,53,54,60,63,68,75,76,82,88,91,93,96).
KRAS mutation and lymph node status (pN category)
Eleven (17%) studies investigated the relationship between KRAS mutation status and presence of lymph node metastases with conflicting results. Five (45%) studies found that KRAS mutant GCs tended to have either no lymph node metastases (46,50,53,54) or significantly fewer lymph node metastases (38). Whereas other studies report that KRAS mutations are more frequent in GCs with lymph node metastases (19,63,68,91,96).
KRAS mutation and histological subtype according to Lauren classification
Seventeen (27%) studies including a total of 2,583 patients investigated the association between KRAS mutation status and histological subtype according to the Lauren classification (19,37,38,40,46,47,56,60,62,63,68,72,75,76,88,91,93). Although 11 (65%) of studies reported a higher incidence of KRAS mutations in intestinal-type GC (see Figure 2), this association did not reach statistical significance in any of the studies (19,37,38,40,56,60,62,68,72,75,91).
KRAS mutation and grade of tumor differentiation
Fifteen (23%) studies investigated the relationship between KRAS mutation and grade of tumor differentiation reporting discordant results. One (7%) study investigating advanced disease found that KRAS mutations were significantly more frequent in histologically differentiated GC (82), three (20%) studies found a higher incidence of KRAS mutations in well-differentiated GCs (47,69,72) whereas nine (60%) studies reported a higher incidence of KRAS mutations in poorly-differentiated GCs (38,46,50,53,54,63,73,75,76). Two studies (13%) found the same incidence of KRAS mutations in well- and poorly-differentiated GC (40,96).
KRAS mutation and survival
Seven (11%) studies investigated the relationship between KRAS mutation status and survival (38,41,62,66,68,76,79), The largest international multicenter study reported a trend towards better survival in patients with a KRAS mutant GC (38). In contrast, subgroup analysis in a different study showed that the median survival of patients with KRAS mutant proximal GCs was significantly shorter (3.5±3.1 months) compared with KRAS wild-type GCs (12.7±0.7 months, P=0.021) (68). The same study found that KRAS mutant intestinal-type GCs had a worse prognosis compared to KRAS wild-type intestinal-type GC, however this difference was not significant on univariate analysis (P=0.098). Similarly, patients with a KRAS mutant GC in the upper third of the stomach may have improved survival over patients with KRAS mutant GC in the middle or distal stomach (76).
KRAS mutation and chemotherapeutic agents
Ten (16%) studies investigated the relationship between KRAS mutations and the use of chemotherapeutic agents (see Table 3). Four studies (40%) did not find any association between KRAS mutation status and progression free survival (PFS) or overall survival (OS) (40,41,62,66), three (30%) studies did not detect any KRAS mutations (42,44,94) and two (20%) studies did not find an association between KRAS mutations and response to chemotherapy (43,90).
KRAS mutation and H. pylori infection
Six (9%) studies have investigated the relationship between H. pylori infection and KRAS mutation status. Three studies reported a higher incidence of KRAS mutations in H. pylori infected GCs, but the difference was not significant or statistical analysis was not performed (47,82,97). In contrast, thirteen (87%) KRAS mutant GCs were found to be H. pylori negative, compared to two H. pylori KRAS mutant GCs (68). One study reported an equal incidence of KRAS mutations in H. pylori positive and negative GCs (75). The study by Hiyama et al. found that KRAS mutations in H. pylori-chronic gastritis were significantly more frequent in patients with GC than those without and in patients with KRAS mutated GC than in KRAS wild-type GC (72).
KRAS mutation and EBV infection
Four (6%) studies investigating a total of 848 GC for KRAS mutation status and EBV infection found no relationship between EBV and KRAS mutation (19,63,68,97).
KRAS mutation status and molecular variables
KRAS mutation and DNA MMR deficiency/MSI (MMR/MSI)
Thirteen (20%) studies investigated the relationship between KRAS mutation status and MMR/MSI with controversial results. One study which included only MSI GC reported that 18% harbored a KRAS mutation (98). Eight (62%) studies reported a higher incidence of MSI in KRAS mutant GCs (39,63,67,70,74), which was significant in three studies (19,75,91). This finding was supported by one study which found that KRAS mutations were more frequent in MMR-deficient GC (38). In contrast, two studies reported that KRAS mutant GC were more frequently microsatellite stable (MSS) (46,68).
KRAS mutation and DNA ploidy
Three (5%) studies investigated the relationship between DNA ploidy and KRAS mutation status. Two investigated DNA ploidy by DNA flow cytometry. One study investigated KRAS mutations in codons 12 and 13 (71), whereas the other study focused on codon 12 (76). Another study investigated DNA ploidy by NGS (19). No associations were reported in any study.
KRAS amplification
Eight (13%) studies investigated KRAS amplification in addition to KRAS mutations with contradictory results. Three studies found that the incidence of KRAS amplification varied between 5% and 9% but was higher than that of KRAS mutation in GC (between 0% and 4%) (59,68,80). In contrast, four studies found that KRAS mutations are more frequent than KRAS amplifications in GC (48,67,87). One study, reported similar frequencies of KRAS amplification (6%) and KRAS mutation (6%) (79). Interestingly, the 5-year survival of patients with a KRAS amplification was worse than that of the patients KRAS mutant GC (HR 3.0, 95% CI: 1.3-7.0). Furthermore, KRAS amplification and KRAS mutation were exclusive. Deng et al. reported that patients with GC with a KRAS amplification had a significantly poorer prognosis, however, as only one KRAS mutation was detected, the relationship between KRAS mutation and prognosis could not be analyzed (59).
The BRAF
BRAF is a member of the RAF family of protein kinases which has three members: ARAF, BRAF and CRAF (100). All RAF proteins share a common structure (101), but BRAF is the only one known to be activated by mutation in human cancer, and therefore the focus of this review (102).
BRAF is also known as v-raf murine sarcoma viral homolog B1 (100) and was discovered in 1988 by Ikawa et al. (103). BRAF is a proto-oncogene and is located on chromosome 7 (7q34) (www.genecards.org, accessed 8th May 2015). BRAF exists in multiple splicing variants, which seem to exhibit tissue specific expression patterns (104).
The BRAF protein is 75 to 100 kDa and has three conserved regions (CR): CR1, CR2 and CR3 (100). CR1 and CR2 are located at the N-terminus and are both regulatory domains, whereas CR3 is a kinase domain and is located at the C-terminus. CR1 is composed of the RAS-binding domain and a cysteine-rich domain binding RAS and membrane phospholipids. CR2 is a serine/threonine rich domain which when phosphorylated can bind regulatory proteins. CR3 is the protein kinase domain which is regulated through phosphorylation (101).
After RAS is activated via extracellular stimuli, it activates BRAF by phosphorylation of two residues in the kinase domain. Activated BRAF phosphorylates and activates MEK1 and MEK2 which then activate MAP kinases ERK1 and ERK2. ERK1/2 activates numerous cytoplasmic and nuclear targets including transcription factors (100).
More than 65 different mutations have been identified in BRAF in human cancer. Most of these mutations are in exon 11 or exon 15 in the catalytic kinase domain (100). The most frequently detected BRAF mutation is a single amino acid substitution (V600E) in exon 15 (105). BRAF is most commonly mutated in melanomas (67%) and CRC (10%) (105,106). Mutant BRAF displays an elevated kinase activity (105) and becomes insensitive to negative feedback mechanisms (107). For a review on BRAF mutations in benign and malignant human tumors, see Michaloglou et al. (108).
BRAF in GC
In total, 22 studies have investigated the incidence of BRAF mutations in GC. Seven (32%) studies screened for BRAF mutations by PCR, followed by direct sequencing (43,61,62,68,70,75,98,109). Other detection methods included denaturing high pressure liquid chromatography, SSCP (39,40,52,93,110), HRMA (42), NGS (46,48,81), amplification-refractory mutation system-PCR, PCR-high resolution melting (50), real-time PCR, immunohistochemistry using a mutation-specific probe (111) or a combination of the above (38,88,112).
Fourteen (64%) studies used FFPE samples (38,39,42,43,48,50,52,61,68,81,88,93,109,111), five (23%) used frozen tissue samples (46,70,75,98,110) and one study used a combination of FFPE and frozen samples (62). Two studies did not report this information (40,112). Excluding the study that performed IHC, ten studies selected areas of tumor with a median tumor cell density of >55%, ranging from >20% to >80% (38,46,48,50,68,70,81,98,109,110). Six studies performed microdissection of the selected area (39,40,52,62,75,93). The remaining five studies did not provide this information (42,43,61,88,112).
All studies investigated the BRAF exon 15 ‘mutation hotspot’ (V600E mutation). Some studies extended their mutation search to exon 11 and other regions of exon 15, or whole genome sequencing. The median BRAF mutation incidence in GC is 0%, ranging from 0% to 12% (38,39,42,43,46,48,50,52,61,62,68,70,75,81,88,93,98,109-112). Only six of the BRAF mutations identified were in V600E of exon 15 (38,40,70,110,112). Six mutations were found in codon 396 and four mutations in codon 608 of exon 15 by Sasao et al. (52). Lee et al. found two mutations in codon 593 and the remaining five mutations were in codon 599 (V599 M) (93) and Okines et al. identified a mutation in V600M and G596D of exon 15 (40).
The highest BRAF mutation incidence (12%) was reported in a Korean study of 17 early and advanced GC using whole-genome sequencing by NGS. The two mutations identified were missense mutations; one was detected in a mixed-type early cancer, the other one in an intestinal-type advanced cancer (46). There has been a single publication that used immunohistochemistry and a mutation specific antibody to detect the mutated BRAF protein as a surrogate for a BRAF mutation. All cases were negative (no evidence suggesting a BRAF mutation) (111).
Due to the low incidence of BRAF mutations no studies have reported a relationship between BRAF mutation status and DNA ploidy or clinicopathological variables. There are three studies that have investigated the relationship between MSI and BRAF mutation. BRAF mutations were not found in any of 37 MSI GC (110) which was confirmed in a study by Wu et al. where the BRAF mutant GC was MSS (70). However, in another study the two BRAF mutant GC were found to be MSI (46).
EGFR pathway in GC
The EGFR pathway is known to be activated in GC (113). When EGFR is bound to its ligand, it triggers homodimerisation and heterodimerisation of the EGFR receptor. This activates a signaling cascade, including MAPK, through effector molecules RAS and RAF (113). Anti-EGFR monoclonal antibodies block ligand-induced binding EGFR tyrosine kinase activation by binding to the extracellular domain of EGFR (114).
Discussion
KRAS and BRAF mutations in GC
The current literature reporting on KRAS and BRAF mutations in GC is very heterogeneous in terms of sample size, patient ethnicity, patient treatment, mutation detection methods, tumor stage and grade of differentiation, as well as other clinicopathological variables.
The majority of studies (70%) investigated the KRAS mutation status in less than 100 patients. Such small studies may not be representative of the GC patient population and thus the patient selection bias may significantly influence any results. Thus, two of the smallest studies with five and seven patients reported some of the highest incidence of KRAS mutations, of 20% and 29%, respectively (41,95). Similarly, for BRAF, the smallest study of 17 patients reported the highest BRAF mutation incidence of 12% (46). Furthermore, twenty-two (34%) studies investigating KRAS mutations deliberately selected subgroups of GC patients to study the KRAS/BRAF mutation status, such as advanced and/or metastatic disease and early disease.
Despite the much higher incidence in the East, the number of studies investigating the relationship between KRAS and BRAF in GC from the East and the West is almost equal. Nevertheless, potential bias due to differences in the histological subtypes (diffuse-type GC is more prevalent in the East), disease stage (GC is diagnosed at an earlier stage in the East) and patient survival (better OS in the East) (115) needs to be considered when comparing study results, particularly in the twenty studies that performed KRAS mutation testing on series from a single center. However, the incidence of KRAS mutations between East and West were comparable and do not seem to be related to the differences in GC incidence (38). Thus, bias due to the patient’s country of origin appears to have no or minimal influence on the incidence of KRAS/BRAF mutations in GC.
An issue that was not addressed in any of the studies included in this review was the potential influence of tumor heterogeneity on the results. Tumor heterogeneity of KRAS and BRAF mutations has been described in CRC suggesting that more than one tumor block should be investigated if possible (116). None of the studies investigating KRAS and/or BRAF mutations in GC seem to have investigated multiple blocks. Studies either did not provide any information or investigated single blocks. Thus, it is impossible to assess whether the incidence of KRAS and/or BRAF mutations in GC is underestimated based on the current literature.
Over ten different methods were used to detect KRAS and/or BRAF mutations in GC. It is known that the sensitivity (ratio of mutant to wild-type) of different methodologies varies between techniques (117), with COLD-PCR having the highest sensitivity (1%) and direct Sanger sequencing having the lowest (10-30%). Despite this low sensitivity, Sanger sequencing is considered the ‘gold standard’ technique due to its ability to detect substitutions, insertion and deletions. The median KRAS mutation incidence in GC appears to be similar irrespective of the detection method and thus, the detection methodology does not appear to affect the incidence of mutations detected in GC.
Several of the studies investigating the use of chemotherapeutic agents in the treatment of GC that also performed KRAS mutation testing, did not provide sufficient information on the type of tissue used for KRAS testing (biopsy/primary resection/recurrent resection/pre- or post-treatment), detection methods used, or codons investigated. Thus it is not possible to accurately interpret the results and make comparisons between such studies. Future studies need to report detailed methodologies in order for conclusions to be drawn from the results.
A recent study suggested that KRAS amplifications contribute to the activation of KRAS in GC (80) and that activation by KRAS amplification may account for the low incidence of KRAS mutations in GC compared to other types of cancer (59). However, the results from studies comparing the incidence and relationship of KRAS mutations (0-10%) and KRAS amplifications (1-9%) in GC remain contradictory (48,59,67,68,79,80,87). Three studies seem to indicate that KRAS amplifications and mutations are mutually exclusive (48,79,80) suggesting a need to screen GC patients for both KRAS mutations and amplifications.
Incidence of KRAS and BRAF mutations—comparison between GC, small bowel and colorectal cancer (CRC)
According to the RASCAL collaborative, the incidence of KRAS mutation in CRC is 38% (118), and a similar incidence has been reported in other studies. Thus, the incidence of KRAS mutations in GC is much lower than in CRC. The incidence of KRAS mutations in small bowel adenocarcinomas seems to vary dramatically from 9-43% based on data from four studies investigating each less than 100 patients and is therefore partly comparable to that of GCs and partly similar to CRCs (51,119-121).
In contrast to GC, in CRC many studies have reported a significant association between BRAF mutation and either deficient MMR status or MSI (106,110,122-126). This could be related to the fact that BRAF mutations are much more frequent in CRC (5-22%) (127) than in GC (0-12%). In adenocarcinomas of the small bowel, the incidence of BRAF mutations is comparable to those reported in GC (119-121). Whereas in CRC KRAS and BRAF mutations appear to be mutually exclusive (128), there are two reports indicating that GC can harbor a KRAS and BRAF mutations simultaneously (48,93). In summary, KRAS mutations in GC are a rare event compared to other cancers of the GI tract. Such differences in the incidence of these mutations between cancers of the GI tract may reflect differences in carcinogenesis.
Although no significant relationship between gender and incidence of KRAS mutations has been reported in GC, KRAS mutations are more frequently reported in males. In addition, the incidence of KRAS mutations is higher in intestinal-type than diffuse-type GC. Both observations may be explained by the fact that the incidence of GC in men is twice as high as in women (2) and that intestinal-type GC is found more frequently in males (129). In CRC, the worldwide incidence is also higher in males but the relative difference is not as prominent as in GC (746,000 new CRC cases per year in males versus 614,000 in females) (2). The relationship between KRAS mutations in CRC and gender is not consistent. One study found a higher incidence of KRAS mutations in females (130), whereas the QUASAR study did not find a difference (122).
Twelve studies investigated the relationship between KRAS mutations and MMR/MSI in GC mostly suggesting a higher incidence of MSI in KRAS mutant GC compared to KRAS wild-type GC. This is in contrast to CRC, where KRAS mutant tumors are found to be less frequent MMR-deficient (118).
In CRC, patients with KRAS wild-type cancer seem to have a better survival (131). Few studies (9%) investigated the relationship between KRAS mutation status and survival in GC and the results do not concur with those from CRC.
KRAS and BRAF mutations and response to anti-EGFR therapy
In CRC, KRAS mutation and BRAF mutation are known predictors of poor response to EGFR targeted agents, such as cetuximab and panitumumab (132) and RAS/BRAF mutation screening is now part of routine clinical diagnosis. In contrast, the predictive value of KRAS and BRAF mutations in GC is far less clear. In vitro, several studies in KRAS wild-type GC cell lines reported sensitivity to EGFR targeting drugs (133-135). Other investigators report that, both KRAS mutant and wild-type GC cell lines were resistant to cetuximab (136). In GC xenografts, apoptosis was only induced in KRAS wild-type tumor cells treated with Cetuximab (136). Cetuximab was shown to reduce tumor volume, dissemination and vascularisation in EGFR-expressing, KRAS wild-type xenografts (133).
To date, the use of anti-EGFR agents (cetuximab and panitumumab) in phase III metastatic GC trials in patients has either showed no difference (137) or poorer survival than the control group (138). In the REAL3 trial, KRAS mutation status did not predict resistance to panitumumab in GC (40).
Due to the low incidence of BRAF mutations in GC, a clinical trial which stratifies GC patients according to their BRAF status is probably not feasible due to the high number of patients that would need to be screened. Although all studies investigated the V600E mutation, three of the studies that also investigated exon 11 and 15 found BRAF mutations other than the hotspot V600E mutation (40,52,93). Thus, there could be an argument for investigating the whole length of the BRAF gene for mutations in GC.
Conclusions
In conclusion, despite the decrease in the incidence, GC remains a major worldwide health problem. KRAS was one of the first oncogenes discovered in GC in 1986. Nevertheless, the current literature on KRAS and BRAF in GC is still limited and very heterogeneous making any comparisons between different studies difficult. However, it appears that the incidence of KRAS mutations in GC is much lower than in CRC, does not differ significantly by ethnicity and that BRAF V600E mutations are very rare in GC. Due to the low incidence and often small studies, many of the published studies did not have enough power to detect a potential relationship between KRAS mutation status and clinicopathological variables including patient survival. Even fewer studies have assessed KRAS amplifications as a mechanism for KRAS activation. So far all clinical studies in unselected metastatic GC have failed to show a significant benefit for EGFR inhibitors. A recent meeting abstract reported the incidence of KRAS mutations in signet ring cell GC is higher (15%) than in other types of GC (139). As the incidence of this histological subtype of GC is increasing, particularly in the West (10) and as this subgroup of GC appears to be highly resistant to standard chemotherapy (140), EGFR targeted therapy in signet ring GC could potentially be a promising treatment option in the future.
Acknowledgements
None.
Footnote
Conflicts of Interest: The authors have no conflicts of interest to declare.
References
- Kamangar F, Dores GM, Anderson WF. Patterns of cancer incidence, mortality, and prevalence across five continents: defining priorities to reduce cancer disparities in different geographic regions of the world. J Clin Oncol 2006;24:2137-50. [PubMed]
- Torre LA, Bray F, Siegel RL, et al. Global cancer statistics, 2012. CA Cancer J Clin 2015;65:87-108. [PubMed]
- Chun N, Ford JM. Genetic testing by cancer site: stomach. Cancer J 2012;18:355-63. [PubMed]
- Plummer M, Franceschi S, Vignat J, et al. Global burden of gastric cancer attributable to Helicobacter pylori. Int J Cancer 2015;136:487-90. [PubMed]
- Bang YJ, Van Cutsem E, Feyereislova A, et al. Trastuzumab in combination with chemotherapy versus chemotherapy alone for treatment of HER2-positive advanced gastric or gastro-oesophageal junction cancer (ToGA): a phase 3, open-label, randomised controlled trial. Lancet 2010;376:687-97. [PubMed]
- Cunningham D, Allum WH, Stenning SP, et al. Perioperative chemotherapy versus surgery alone for resectable gastroesophageal cancer. N Engl J Med 2006;355:11-20. [PubMed]
- Hu B, El Hajj N, Sittler S, et al. Gastric cancer: Classification, histology and application of molecular pathology. J Gastrointest Oncol 2012;3:251-61. [PubMed]
- Lauren P. The two histological main types of gastric carcinoma: diffuse and so called intestinal-type carcinoma. Acta Pathol Microbiol Scand 1965;64:31-49. [PubMed]
- Kong X, Wang JL, Chen HM, et al. Comparison of the clinicopathological characteristics of young and elderly patients with gastric carcinoma: a meta analysis. J Surg Oncol 2012;106:346-52. [PubMed]
- Wu H, Rusiecki JA, Zhu K, et al. Stomach carcinoma incidence patterns in the United States by histologic type and anatomic site. Cancer Epidemiol. Biomarkers Prev 2009;18:1945-52. [PubMed]
- Qiu MZ, Cai MY, Zhang DS, et al. Clinicopathological characteristics and prognostic analysis of Lauren classification in gastric adenocarcinoma in China. J Transl Med 2013;11:58. [PubMed]
- Kwon SJ. Evaluation of the 7th UICC TNM Staging System of Gastric Cancer. J Gastric Cancer 2011;11:78-85. [PubMed]
- Washington K. 7th edition of the AJCC cancer staging manual: stomach. Ann Surg Oncol 2010;17:3077-9.
- Japanese Gastric Cancer Association. Japanese classification of gastric carcinoma: 3rd English edition. Gastric Cancer 2011;14:101-12. [PubMed]
- Shi J, Qu YP, Hou P. Pathogenetic mechanisms in gastric cancer. World J Gastroenterol 2014;20:13804-19. [PubMed]
- Velho S, Fernandes MS, Leite M, et al. Causes and consequences of microsatellite instability in gastric carcinogenesis. World J Gastroenterol 2014;20:16433-42. [PubMed]
- Oki E, Hisamatsu Y, Ando K, et al. Clinical aspect and molecular mechanism of DNA aneuploidy in gastric cancers. J Gastroenterol 2012;47:351-8. [PubMed]
- Gulley ML. Genomic assays for Epstein-Barr virus-positive gastric adenocarcinoma. Exp Mol Med 2015;47:e134. [PubMed]
- Cancer Genome Atlas Research Network. Comprehensive molecular characterization of gastric adenocarcinoma. Nature 2014;513:202-9. [PubMed]
- Tan P, Yeoh KG. Genetics and Molecular Pathogenesis of Gastric Adenocarcinoma. Gastroenterology 2015;149:1153-62. e3.
- Chang EH, Gonda MA, Ellis RW, et al. Human genome contains four genes homologous to transforming genes of Harvey and Kirsten murine sarcoma viruses. Proc Natl Acad Sci U S A 1982;79:4848-52. [PubMed]
- Shimizu K, Goldfarb M, Perucho M, et al. Isolation and preliminary characterization of the transforming gene of a human neuroblastoma cell line. Proc Natl Acad Sci U S A 1983;80:383-7. [PubMed]
- Lowy DR, Willumsen BM. Function and regulation of ras. Annu Rev Biochem 1993;62:851-91. [PubMed]
- Pylayeva-Gupta Y, Grabocka E, Bar-Sagi D. RAS oncogenes: weaving a tumorigenic web. Nat Rev Cancer 2011;11:761-74. [PubMed]
- Bos JL. ras oncogenes in human cancer: A review. Cancer Res 1989;49:4682-9. [PubMed]
- Charette N, Vandeputte C, Starkel P. Ras in digestive oncology: from molecular biology to clinical implications. Curr Opin Oncol 2014;26:454-61. [PubMed]
- Barbacid M. ras genes. Annu Rev Biochem 1987;56:779-827. [PubMed]
- Jancík S, Drábek J, Radzioch D, et al. Clinical relevance of KRAS in human cancers. J Biomed Biotechnol 2010;2010:150960.
- Malumbres M, Barbacid M. RAS oncogenes: the first 30 years. Nat Rev Cancer 2003;3:459-65. [PubMed]
- Malumbres M, Pellicer A. RAS pathways to cell cycle control and cell transformation. Front Biosci 1998;3:d887-912. [PubMed]
- Moodie SA, Willumsen BM, Weber MJ, et al. Complexes of Ras.GTP with Raf-1 and mitogen-activated protein kinase kinase. Science 1993;260:1658-61. [PubMed]
- Rodriguez-Viciana P, Warne PH, Dhand R, et al. Phosphatidylinositol-3-OH kinase as a direct target of Ras. Nature 1994;370:527-32. [PubMed]
- Chetty R, Govender D. Gene of the month: KRAS. J Clin Pathol 2013;66:548-50. [PubMed]
- Prior IA, Lewis PD, Mattos C. A Comprehensive Survey of Ras Mutations in Cancer. Cancer Res 2012;72:2457-67. [PubMed]
- Serrano M, Lin AW, McCurrach ME, et al. Oncogenic ras provokes premature cell senescence associated with accumulation of p53 and p16INK4a. Cell 1997;88:593-602. [PubMed]
- Bos JL, Vries MV, Marshall CJ, et al. A human gastric carcinoma contains a single mutated and an amplified normal allele of the Ki-ras oncogene. Nucleic Acids Res 1986;14:1209-17. [PubMed]
- Yoo J, Park SY, Robinson RA, et al. ras gene mutations and expression of ras signal transduction mediators in gastric adenocarcinomas. Arch Pathol Lab Med 2002;126:1096-100. [PubMed]
- van Grieken NC, Aoyama T, Chambers PA, et al. KRAS and BRAF mutations are rare and related to DNA mismatch repair deficiency in gastric cancer from the East and the West: results from a large international multicentre study. Br J Cancer 2013;108:1495-501. [PubMed]
- Gylling A, Abdel-Rahman WM, Juhola M, et al. Is gastric cancer part of the tumour spectrum of hereditary non-polyposis colorectal cancer? A molecular genetic study. Gut 2007;56:926-33. [PubMed]
- Okines AF, Gonzalez de Castro D, Cunningham D, et al. Biomarker analysis in oesophagogastric cancer: Results from the REAL3 and TransMAGIC trials. Eur J Cancer 2013;49:2116-25. [PubMed]
- Rohrberg KS, Pappot H, Lassen U, et al. Biomarkers in tissue from patients with upper gastrointestinal cancers treated with erlotinib and bevacizumab. Cancer Biol Ther 2011;11:732-9. [PubMed]
- Moehler M, Mueller A, Hartmann JT, et al. An open-label, multicentre biomarker-oriented AIO phase II trial of sunitinib for patients with chemo-refractory advanced gastric cancer. Eur J Cancer 2011;47:1511-20. [PubMed]
- Pinto C, Di Fabio F, Barone C, et al. Phase II study of cetuximab in combination with cisplatin and docetaxel in patients with untreated advanced gastric or gastro-oesophageal junction adenocarcinoma (DOCETUX study). Br J Cancer 2009;101:1261-8. [PubMed]
- Han SW, Oh DY, Im SA, et al. Phase II study and biomarker analysis of cetuximab combined with modified FOLFOX6 in advanced gastric cancer. Br J Cancer 2009;100:298-304. [PubMed]
- Sakurai S, Sano T, Maeshima A, et al. Gastric adenoma-carcinoma sequence with special reference to p53 and Ki-ras gene alterations. Virchows Arch 1995;427:119-24. [PubMed]
- Kim TM, Jung SH, Kim MS, et al. The mutational burdens and evolutionary ages of early gastric cancers are comparable to those of advanced gastric cancers. J Pathol 2014;234:365-74. [PubMed]
- Hongyo T, Buzard GS, Palli D, et al. Mutations of the K-ras and p53 genes in gastric adenocarcinomas from a high-incidence region around Florence, Italy. Cancer Res 1995;55:2665-72. [PubMed]
- Ali SM, Sanford EM, Klempner SJ, et al. Prospective comprehensive genomic profiling of advanced gastric carcinoma cases reveals frequent clinically relevant genomic alterations and new routes for targeted therapies. Oncologist 2015;20:499-507. [PubMed]
- Lordick F, Luber B, Lorenzen S, et al. Cetuximab plus oxaliplatin/leucovorin/5-fluorouracil in first-line metastatic gastric cancer: a phase II study of the Arbeitsgemeinschaft Internistische Onkologie (AIO). Br J Cancer 2010;102:500-5. [PubMed]
- Lu W, Wei H, Li M, et al. Identification of KRAS and PIK3CA but not BRAF mutations in patients with gastric cancer. Mol Med Rep 2015;12:1219-24. [PubMed]
- Arber N, Shapira I, Ratan J, et al. Activation of c-K-ras mutations in human gastrointestinal tumors. Gastroenterology 2000;118:1045-50. [PubMed]
- Sasao S, Hiyama T, Tanaka S, et al. Clinicopathologic and genetic characteristics of gastric cancer in young male and female patients. Oncol Rep 2006;16:11-5. [PubMed]
- Liu ZM, Liu LN, Li M, et al. Mutation detection of KRAS by high-resolution melting analysis in Chinese with gastric cancer. Oncol Rep 2009;22:515-20. [PubMed]
- Liu Z, Liu L, Li M, et al. Epidermal growth factor receptor mutation in gastric cancer. Pathology 2011;43:234-8. [PubMed]
- Peng N, Zhao X. Comparison of mutations in lung, colorectal and gastric cancer. Oncol Lett 2014;8:561-5. [PubMed]
- Miki H, Ohmori M, Perantoni AO, et al. K-ras activation in gastric epithelial tumors in Japanese. Cancer Lett 1991;58:107-13. [PubMed]
- Nagata Y, Abe M, Kobayashi K, et al. Glycine to aspartic acid mutations at codon 13 of the c-Ki-ras gene in human gastrointestinal cancers. Cancer Res 1990;50:480-2. [PubMed]
- Victor T, Du Toit R, Jordaan AM, et al. No evidence for point mutations in codons 12, 13, and 61 of the ras gene in a high-incidence area for esophageal and gastric cancers. Cancer Res 1990;50:4911-4. [PubMed]
- Deng N, Goh LK, Wang H, et al. A comprehensive survey of genomic alterations in gastric cancer reveals systematic patterns of molecular exclusivity and co-occurrence among distinct therapeutic targets. Gut 2012;61:673-84. [PubMed]
- Palacio-Rúa KA, Isaza-Jiménez LF, Ahumada-Rodríguez E, et al. Genetic analysis in APC, KRAS, and TP53 in patients with stomach and colon cancer. Rev Gastroenterol Mex 2014;79:79-89. [PubMed]
- Matsubara A, Sekine S, Kushima R, et al. Frequent GNAS and KRAS mutations in pyloric gland adenoma of the stomach and duodenum. J Pathol 2013;229:579-87. [PubMed]
- Takahashi N, Yamada Y, Taniguchi H, et al. Clinicopathological features and prognostic roles of KRAS, BRAF, PIK3CA and NRAS mutations in advanced gastric cancer. BMC Res Notes 2014;7:271. [PubMed]
- Betge J, Pollheimer MJ, Schlemmer A, et al. Gastric cancer and concomitant renal cancer: a systematic immunohistochemical and molecular analysis. Oncol Rep 2011;26:567-75. [PubMed]
- Tajima Y, Yamazaki K, Makino R, et al. Differences in the histological findings, phenotypic marker expressions and genetic alterations between adenocarcinoma of the gastric cardia and distal stomach. Br J Cancer 2007;96:631-8. [PubMed]
- Tajima Y, Yamazaki K, Makino R, et al. Gastric and intestinal phenotypic marker expression in early differentiated-type tumors of the stomach: clinicopathologic significance and genetic background. Clin Cancer Res 2006;12:6469-79. [PubMed]
- Park SR, Kook MC, Choi IJ, et al. Predictive factors for the efficacy of cetuximab plus chemotherapy as salvage therapy in metastatic gastric cancer patients. Cancer Chemother Pharmacol 2010;65:579-87. [PubMed]
- Cristescu R, Lee J, Nebozhyn M, et al. Molecular analysis of gastric cancer identifies subtypes associated with distinct clinical outcomes. Nat Med 2015;21:449-56. [PubMed]
- Warneke VS, Behrens HM, Haag J, et al. Prognostic and putative predictive biomarkers of gastric cancer for personalized medicine. Diagn Mol Pathol 2013;22:127-37. [PubMed]
- Kihana T, Tsuda H, Hirota T, et al. Point mutation of c-Ki-ras oncogene in gastric adenoma and adenocarcinoma with tubular differentiation. Jpn J Cancer Res 1991;82:308-14. [PubMed]
- Wu M, Semba S, Oue N, et al. BRAF/K-ras mutation, microsatellite instability, and promoter hypermethylation of hMLH1/MGMT in human gastric carcinomas. Gastric Cancer 2004;7:246-53. [PubMed]
- Russo A, Bazan V, Migliavacca M, et al. DNA aneuploidy and high proliferative activity but not K-ras-2 mutations as independent predictors of clinical outcome in operable gastric carcinoma. Cancer 2001;92:294-302. [PubMed]
- Hiyama T, Haruma K, Kitadai Y, et al. K-ras mutation in helicobacter pylori-associated chronic gastritis in patients with and without gastric cancer. Int J Cancer 2002;97:562-6. [PubMed]
- Koshiba M, Ogawa O, Habuchi T, et al. Infrequent ras mutation in human stomach cancers. Jpn J Cancer Res 1993;84:163-7. [PubMed]
- Lee JH, Abraham SC, Kim HS, et al. Inverse relationship between APC gene mutation in gastric adenomas and development of adenocarcinoma. Am J Pathol 2002;161:611-8. [PubMed]
- Zhao W, Chan TL, Chu KM, et al. Mutations of BRAF and KRAS in gastric cancer and their association with microsatellite instability. Int J Cancer 2004;108:167-9. [PubMed]
- Lee KH, Lee JS, Suh C, et al. Clinicopathologic significance of the K-ras gene codon 12 point mutation in stomach cancer. An analysis of 140 cases. Cancer 1995;75:2794-801. [PubMed]
- Saxena A, Shukla SK, Prasad KN, et al. Analysis of p53, K-ras gene mutation & Helicobacter pylori infection in patients with gastric cancer & peptic ulcer disease at a tertiary care hospital in north India. Indian J Med Res 2012;136:664-70. [PubMed]
- Kimura K, Nagasaka T, Hoshizima N, et al. No duplicate KRAS mutation is identified on the same allele in gastric or colorectal cancer cells with multiple KRAS mutations. J Int Med Res 2007;35:450-7. [PubMed]
- Qian Z, Zhu G, Tang L, et al. Whole genome gene copy number profiling of gastric cancer identifies PAK1 and KRAS gene amplification as therapy targets. Genes Chromosomes Cancer 2014;53:883-94. [PubMed]
- Mita H, Toyota M, Aoki F, et al. A novel method, digital genome scanning detects KRAS gene amplification in gastric cancers: involvement of overexpressed wild-type KRAS in downstream signaling and cancer cell growth. BMC Cancer 2009;9:198. [PubMed]
- Kim S, Lee J, Hong ME, et al. High-throughput sequencing and copy number variation detection using formalin fixed embedded tissue in metastatic gastric cancer. PLoS One 2014;9:e111693. [PubMed]
- Yashiro M, Nishioka N, Hirakawa K. K-ras mutation influences macroscopic features of gastric carcinoma. J Surg Res 2005;124:74-8. [PubMed]
- Capella G, Cronauer-Mitra S, Pienado MA, et al. Frequency and spectrum of mutations at codons 12 and 13 of the c-K-ras gene in human tumors. Environ Health Perspect 1991;93:125-31. [PubMed]
- Craanen ME, Blok P, Top B, et al. Absence of ras gene mutations in early gastric carcinomas. Gut 1995;37:758-62. [PubMed]
- Oliveira C, Velho S, Domingo E, et al. Concomitant RASSF1A hypermethylation and KRAS/BRAF mutations occur preferentially in MSI sporadic colorectal cancer. Oncogene 2005;24:7630-4. [PubMed]
- Hosoi H, Hirosawa S, Yamamoto K, et al. Using Biopsy Materials to Detect Mutations in Gastrointestinal Tumors. J Clin Gastroenterol 1995;20:272-6. [PubMed]
- Yoda Y, Takeshima H, Niwa T, et al. Integrated analysis of cancer-related pathways affected by genetic and epigenetic alterations in gastric cancer. Gastric Cancer 2015;18:65-76. [PubMed]
- Kim IJ, Park JH, Kang H, et al. Mutational analysis of BRAF and K-ras in gastric cancers: absence of BRAF mutations in gastric cancers. Hum Genet 2003;114:118-20. [PubMed]
- Ranzani GN, Renault B, Pellegata NS, et al. Loss of heterozygosity and K-ras gene mutations in gastric cancer. Hum Genet 1993;92:244-9. [PubMed]
- Richards D, Kocs DM, Spira AI, et al. Results of docetaxel plus oxaliplatin (DOCOX) +/- cetuximab in patients with metastatic gastric and/or gastroesophageal junction adenocarcinoma: results of a randomised Phase 2 study. Eur J Cancer 2013;49:2823-31. [PubMed]
- Brennetot C, Duval A, Hamelin R, et al. Frequent ki-ras mutations in gastric tumors of the MSI phenotype. Gastroenterology 2003;125:1282-3. [PubMed]
- Hao Y, Zhang J, Lu Y, et al. The role of ras gene mutation in gastric cancer and precancerous lesions. J Tongji Med Univ 1998;18:141-4. [PubMed]
- Lee SH, Lee JW, Soung YH, et al. BRAF and KRAS mutations in stomach cancer. Oncogene 2003;22:6942-5. [PubMed]
- Wöll E, Greil R, Eisterer W, et al. Oxaliplatin, irinotecan and cetuximab in advanced gastric cancer. A multicenter phase II trial (Gastric-2) of the Arbeitsgemeinschaft Medikamentose Tumortherapie (AGMT). Anticancer Res 2011;31:4439-43. [PubMed]
- Iwaya T, Maesawa C, Nishizuka S, et al. Infrequent frameshift mutations of polynucleotide repeats in multiple primary cancers affecting the esophagus and other organs. Genes Chromosomes Cancer 1998;23:317-22. [PubMed]
- Kim JG, Takeshima H, Niwa T, et al. Comprehensive DNA methylation and extensive mutation analyses reveal an association between the CpG island methylator phenotype and oncogenic mutations in gastric cancers. Cancer Lett 2013;330:33-40. [PubMed]
- Kusano M, Toyota M, Suzuki H, et al. Genetic, epigenetic, and clinicopathologic features of gastric carcinomas with the CpG island methylator phenotype and an association with Epstein-Barr virus. Cancer 2006;106:1467-79. [PubMed]
- Corso G, Velho S, Paredes J, et al. Oncogenic mutations in gastric cancer with microsatellite instability. Eur J Cancer 2011;47:443-51. [PubMed]
- Chen HC, Chen HJ, Khan MA, et al. Genetic mutations of p53 and k-ras in gastric carcinoma patients from Hunan, China. Tumour Biol 2011;32:367-73. [PubMed]
- Rahman MA, Salajegheh A, Smith RA, et al. B-Raf mutation: a key player in molecular biology of cancer. Exp Mol Pathol 2013;95:336-42. [PubMed]
- Roskoski R Jr. RAF protein-serine/threonine kinases: structure and regulation. Biochem Biophys Res Commun 2010;399:313-7. [PubMed]
- Dhomen N, Marais R. New insight into BRAF mutations in cancer. Curr Opin Genet Dev 2007;17:31-9. [PubMed]
- Ikawa S, Fukui M, Ueyama Y, et al. B-raf, a new member of the raf family, is activated by DNA rearrangement. Mol Cell Biol 1988;8:2651-4. [PubMed]
- Barnier JV, Papin C, Eychene A, et al. The mouse B-raf gene encodes multiple protein isoforms with tissue-specific expression. J Biol Chem 1995;270:23381-9. [PubMed]
- Davies H, Bignell GR, Cox C, et al. Mutations of the BRAF gene in human cancer. Nature 2002;417:949-54. [PubMed]
- Rajagopalan H, Bardelli A, Lengauer C, et al. Tumorigenesis: RAF/RAS oncogenes and mismatch-repair status. Nature 2002;418:934. [PubMed]
- Tsavachidou D, Coleman ML, Athanasiadis G, et al. SPRY2 is an inhibitor of the ras/extracellular signal-regulated kinase pathway in melanocytes and melanoma cells with wild-type BRAF but not with the V599E mutant. Cancer Res 2004;64:5556-9. [PubMed]
- Michaloglou C, Vredeveld LC, Mooi WJ, et al. BRAF(E600) in benign and malignant human tumours. Oncogene 2008;27:877-95. [PubMed]
- Luber B, Deplazes J, Keller G, et al. Biomarker analysis of cetuximab plus oxaliplatin/leucovorin/5-fluorouracil in first-line metastatic gastric and oesophago-gastric junction cancer: results from a phase II trial of the Arbeitsgemeinschaft Internistische Onkologie (AIO). BMC Cancer 2011;11:509. [PubMed]
- Oliveira C, Pinto M, Duval A, et al. BRAF mutations characterize colon but not gastric cancer with mismatch repair deficiency. Oncogene 2003;22:9192-6. [PubMed]
- Preusser M, Berghoff AS, Capper D, et al. No Evidence for BRAF-V600E Mutations in Gastroeosophageal Tumors: Results From a High-throughput Analysis of 534 Cases Using a Mutation-specific Antibody. Appl Immunohistochem Mol Morphol 2013;21:426-30. [PubMed]
- Machnicki MM, Glodkowska-Mrowka E, Lewandowski T, et al. ARMS-PCR for detection of BRAF V600E hotspot mutation in comparison with Real-Time PCR-based techniques. Acta Biochim Pol 2013;60:57-64. [PubMed]
- Rivera F, Vega-Villegas ME, Lopez-Brea MF. Cetuximab, its clinical use and future perspectives. Anticancer Drugs 2008;19:99-113. [PubMed]
- Ciardiello F, Tortora G. EGFR antagonists in cancer treatment. N Engl J Med 2008;358:1160-74. [PubMed]
- Bickenbach K, Strong VE. Comparisons of Gastric Cancer Treatments: East vs. West. J Gastric Cancer 2012;12:55-62. [PubMed]
- Richman SD, Chambers P, Seymour MT, et al. Intra-tumoral heterogeneity of KRAS and BRAF mutation status in patients with advanced colorectal cancer (aCRC) and cost-effectiveness of multiple sample testing. Anal Cell Pathol (Amst) 2011;34:61-6. [PubMed]
- Anderson SM. Laboratory methods for KRAS mutation analysis. Expert Rev Mol Diagn 2011;11:635-42. [PubMed]
- Andreyev HJ, Norman AR, Cunningham D, et al. Kirsten ras mutations in patients with colorectal cancer: the multicenter "RASCAL" study. J Natl Cancer Inst 1998;90:675-84. [PubMed]
- Matsubara A, Ogawa R, Suzuki H, et al. Activating GNAS and KRAS mutations in gastric foveolar metaplasia, gastric heterotopia, and adenocarcinoma of the duodenum. Br J Cancer 2015;112:1398-404. [PubMed]
- Kumagai R, Kohashi K, Takahashi S, et al. Mucinous phenotype and CD10 expression of primary adenocarcinoma of the small intestine. World J Gastroenterol 2015;21:2700-10. [PubMed]
- Aparicio T, Svrcek M, Zaanan A, et al. Small bowel adenocarcinoma phenotyping, a clinicobiological prognostic study. Br J Cancer 2013;109:3057-66. [PubMed]
- Hutchins G, Southward K, Handley K, et al. Value of mismatch repair, KRAS, and BRAF mutations in predicting recurrence and benefits from chemotherapy in colorectal cancer. J Clin Oncol 2011;29:1261-70. [PubMed]
- Calistri D, Rengucci C, Seymour I, et al. KRAS, p53 and BRAF gene mutations and aneuploidy in sporadic colorectal cancer progression. Cell Oncol 2006;28:161-6. [PubMed]
- Samowitz WS, Sweeney C, Herrick J, et al. Poor survival associated with the BRAF V600E mutation in microsatellite-stable colon cancers. Cancer Res 2005;65:6063-9. [PubMed]
- Wang L, Cunningham JM, Winters JL, et al. BRAF mutations in colon cancer are not likely attributable to defective DNA mismatch repair. Cancer Res 2003;63:5209-12. [PubMed]
- Kadowaki S, Kakuta M, Takahashi S, et al. Prognostic value of KRAS and BRAF mutations in curatively resected colorectal cancer. World J Gastroenterol 2015;21:1275-83. [PubMed]
- Chen D, Huang JF, Liu K, et al. BRAFV600E mutation and its association with clinicopathological features of colorectal cancer: a systematic review and meta-analysis. PLoS One 2014;9:e90607. [PubMed]
- Cunningham D, Atkin W, Lenz HJ, et al. Colorectal cancer. Lancet 2010;375:1030-47. [PubMed]
- Derakhshan MH, Liptrot S, Paul J, et al. Oesophageal and gastric intestinal-type adenocarcinomas show the same male predominance due to a 17 year delayed development in females. Gut 2009;58:16-23. [PubMed]
- Tong JH, Lung RW, Sin FM, et al. Characterization of rare transforming KRAS mutations in sporadic colorectal cancer. Cancer Biol Ther 2014;15:768-76. [PubMed]
- Andreyev HJ, Norman AR, Cunningham D, et al. Kirsten ras mutations in patients with colorectal cancer: the 'RASCAL II' study. Br J Cancer 2001;85:692-6. [PubMed]
- Heinemann V, Stintzing S, Kirchner T, et al. Clinical relevance of EGFR- and KRAS-status in colorectal cancer patients treated with monoclonal antibodies directed against the EGFR. Cancer Treat Rev 2009;35:262-71. [PubMed]
- Hotz B, Keilholz U, Fusi A, et al. In vitro and in vivo antitumor activity of cetuximab in human gastric cancer cell lines in relation to epidermal growth factor receptor (EGFR) expression and mutational phenotype. Gastric Cancer 2012;15:252-64. [PubMed]
- Heindl S, Eggenstein E, Keller S, et al. Relevance of MET activation and genetic alterations of KRAS and E-cadherin for cetuximab sensitivity of gastric cancer cell lines. J Cancer Res Clin Oncol 2012;138:843-58. [PubMed]
- Kneissl J, Keller S, Lorber T, et al. Association of amphiregulin with the cetuximab sensitivity of gastric cancer cell lines. Int J Oncol 2012;41:733-44. [PubMed]
- Shi M, Shi HL, Ji J, et al. Cetuximab Inhibits Gastric Cancer Growth in vivo, Independent of KRAS Status. Curr Cancer Drug Targets 2014;14:217-24. [PubMed]
- Lordick F, Kang YK, Chung HC, et al. Capecitabine and cisplatin with or without cetuximab for patients with previously untreated advanced gastric cancer (EXPAND): a randomised, open-label phase 3 trial. Lancet Oncol 2013;14:490-9. [PubMed]
- Waddell T, Chau I, Cunningham D, et al. Epirubicin, oxaliplatin, and capecitabine with or without panitumumab for patients with previously untreated advanced oesophagogastric cancer (REAL3): a randomised, open-label phase 3 trial. Lancet Oncol 2013;14:481-9. [PubMed]
- Liu B, Wei J, Wu N, et al. Evaluation of driver mutations involving in RAS-RAF/PI3K-mToR pathway in gastric signet ring cell carcinoma. J Clin Oncol 2015;33:abstr e15077.
- Piessen G, Messager M, Le Malicot K, et al. Phase II/III multicentre randomised controlled trial evaluating a strategy of primary surgery and adjuvant chemotherapy versus peri-operative chemotherapy for resectable gastric signet ring cell adenocarcinomas - PRODIGE 19 - FFCD1103 - ADCI002. BMC Cancer 2013;13:281. [PubMed]