Histamine and histamine receptor regulation of gastrointestinal cancers
Abstract: Histamine is a neurotransmitter released throughout the body that regulates multiple physiological responses. Primarily histamine is acknowledged for its role in inflammatory reactions to foreign pathogens that enter the body. Aside from inflammatory responses, histamine expression and synthesis has been detected in various cancer cell lines and multiple malignancies. Through experimentation histamine has demonstrated its ability to manage proliferation and angiogenesis in these cancerous cells, in either a positive or inhibitory manner. Regulation of angiogenesis and proliferation have been proven to be carried out by the stimulation or inhibition of numerous pathways and secondary response elements, such as VEGFA/C, IP3/Ca2+, G-proteins, cAMP, and many more. The activation of these different response pathways is linked to the binding of ligands to the histamine receptors H1-H4HR. These receptors exhibit various effects dependent on whether it binds an agonist, antagonist, or its specific ligand, histamine. In cancer cell lines and different tumor cells the binding of these different compounds has shown to be one of the main components in exerting proliferative or antiproliferative changes in the microenvironment. It is also known that the histamine receptors have varying degrees of expression in different forms of cancer, and this expression can impact the tumor in various ways. This clearly indicates the significance of histamine receptors in cancer formation, and one of the aims of this review is to cover this topic concisely and in depth. Histamine is produced from numerous cells such as basophils and mast cells and is synthesized from the enzyme histidine decarboxylase (HDC). In this review we will prominently discuss the function of mast cells and HDC in histamine expression in various gastrointestinal carcinomas. We also briefly discuss current studies to support these claims. In this review we hope to give the reader a clear and comprehensible overview of histamine in various gastrointestinal cancers, and how its regulation can affect the cancer cells in varying ways.
Key words: Histamine; gastrointestinal cancers; histidine decarboxylase
Histamine and HDC
Histamine is a biogenic amine that is released throughout the entire body of an organism via paracrine and/or autocrine mechanisms (1-5). Recent studies have shown that the highest concentration of histamine is present in the stomach, lymph nodes, and thymus (6). The lowest concentrations of histamine are found in the brain, liver, lung, and intestines (6). It is known that histamine is induced and readily made available in rapidly growing tissues (7). Within these tissues, intracellular histamine is released from mast cells, basophils and other types of cells (8,9). Histamine can also be catalyzed from L-histidine by the enzyme L-histidine decarboxylase (HDC) (10,11). HDC is able to regulate various physiological and pathological responses, and it is mainly found in the mast cells, skin cells, platelets, basophils, immature myeloid cells (IMC’s) and gastric enterochromaffin-like (ECL) cells (12,13). Studies have shown that HDC is expressed in neuroendocrine tumors, most malignant cell lines and experimental tumors (10,14). In rat hypothalamus and lung samples, there is evidence that HDC is activated by protein kinase A (12,15). Another study has demonstrated how the effects of the HDC enzyme activity can be regulated through its phosphorylation (12,16). The peptide hormone, gastrin, transcriptionally regulates HDC activity, but the possibility of HDC activation through post-translational modifications by other proteins is conceivable as well (12). In adult animals, HDC is detected in the liver, corpus and pancreas, with the liver containing the highest concentration and the gastric corpus and pancreas containing the lowest concentration of this enzyme (12).
Histamine receptor action
Histamine is an important pleiotropic chemical that regulates various pathophysiological functions such as cellular invasion, migration, differentiation, apoptosis, angiogenesis, and different immune responses (1,12,17). Histamine mediates these different cellular responses through its receptors, H1-H4HR, and the expression of these different receptors can be altered in multiple diseases, and varies between different types of tissues (18,19). H1HR is a G-protein coupled receptor that exhibits its effects through the activation of Gαq, a subfamily of heterotameric G-proteins (20,21). After activating a Gαq protein the IP3/Ca2+ cascade mechanism is then activated and is able to exert its effects (20,21). H1HR has been located in the brain, smooth muscle, gastrointestinal tract, endothelial cells, and lymphocytes (4,22). Functions of H1HR, dependent on location, include smooth muscle contractions, changes in vascular permeability, increase in heart rate, increase in nasal mucus production, widened pulse pressure, and T-cell neutrophil and eosinophil chemotaxis (4,23-26). H2HR activates Gαs that stimulates cAMP, which leads to different downstream cascade effects (20). H2HR has been found in areas of the brain, gastric cells, and cardiac tissue (4,20,27,28). Actions of H2HR consist of increases in venular permeability, increase in gastric acid secretion, airway mucus production, and inhibition of neutrophil and eosinophil influx (29,30). The role of the H3HR signaling transduction pathways still remains undefined, and their downstream effects are largely up for speculation (4,31-33). Some research into the notion that H3HR inhibits adenylyl cyclase activity in different cells and tissues have all failed, which leads to the assumption that H3HR preferentially couples to Gαi/o proteins (4,32,33). H3HR has been located in the brain and endocrine cells in the gastrointestinal (GI) mucosa (31,34). H4HR signaling studied in transfected H4HR transfected cells has shown that its activation will inactivate cAMP production through adenylyl cyclase, and it has been postulated that this is achieved through the involvement of Gαi/o proteins (35-38). H4HRs are found within the GI tract, epithelial cells, and at low levels in immune cells (1,19). This receptor has experimentally proven to modulate Th2 responses, act as a chemoattractant for mouse bone marrow derived mast cells, changes in Ca2+ influx, and decreases the amount of infiltration of eosinophils and lymphocytes in mice lung inflammation (39,40).
Development of cancer
The growth and development of tumors in humans is a multistep process that arises from various genetic and epigenetic alterations that transform normal cells into malignant cells (14). Multiple molecules are known to be a factor in tumorigenesis, but one of the most studied molecules is histamine (14,41,42). Research has shown that abnormal inflammatory responses, mainly persistent ones, promote invasion, formation, progression and angiogenic activity of some tumors (14). Developments of these cancerous processes are mostly seen in tissues adjacent to areas of prolonged inflammation (14). Histamine can have different effects on cancerous cells depending on the tumor cell features (43). In some cases histamine has shown to increase cell proliferation and tumor growth (18,43). These instances show histamine as being either an autocrine or paracrine growth factor that stimulates the proliferation rate of various normal and malignant tissues (43,44). Decreases in cellular proliferation and antitumor responses can also be regulated through histamines downstream effects (7). Histamine released by mast cells is commonly known to induce tumor proliferation and immunosuppression through the expression patterns of its assorted histamine receptors (45,46). Many human cell lines derived from neoplasias, and some tumoral tissues as well, express numerous histamine receptors that aid in cellular proliferation, findings demonstrating the ability for histamine to act as a growth factor (14,44,47-51). Some research has shown that these different histamine receptors are expressed in various malignant cell types (43,48).
Mast cells
Mast cells are an important component of the tumor microenvironment where it has a complex role that is still being elucidated (45). Mast cells have been proven to promote cancer growth through angiogenesis, tissue remodeling, and manipulation of immune responses; these processes can be mediated by protease-activated receptors, mitogen activated protein kinases, prostaglandins, and histamine (45). Mast cells have also shown to exert anticancer actions such as growth inhibition, immunologic stimulation, inhibition of apoptosis, and decrease in cell mobility (45). Mediators released by mast cells have proven to induce either pro-cancer or anti-cancer effects dependent on their concentration, present cofactors, and location of where they are being secreted (45). Just like histamine, mast cells can play different roles in different cancer cells depending on tumor cell features (43). Presence and concentration of HDC in tumors can also have an effect on tumor growth (12,48). When stimulated, HDC has shown to contribute to growth and proliferation (12,48). HDC over expression has been shown in various forms of cancer such as colon, breast, stomach, and lung (7). This over expression of HDC then increases the amount of cellular histamine, which can lead to pro-proliferative effects (7,14). Inhibition of HDC in rodent tumor samples has resulted in antitumor responses (14).
Gastric cancer
Gastric cancer is the most common cause of cancer related deaths around the world with an average 700,000 deaths a year (52,53). The amount of patients diagnosed with gastric adenocarcinoma has decreased in the past decade, but it is still a devastating form of cancer that requires future research (54-56). Gastric cancer tends to form sporadically, but environmental factors such as smoking, alcohol consumption, and dietary habits can lead to the eventual development of cancerous cells (52). It has been demonstrated that some stomach diseases, such as gastric epithelial dysplasia, can act as precursors to gastric cancer (57). There is evidence that some human gastric cancer cell lines are stimulated by histamine (58). Experimental evidence has proven that this histamine is synthesized by and released from ECL (enterochromaffin-like) cells (12,59). ECL cells will release histamine in response to acute gastrin stimulation that eventually leads to depletion of histamine within ECL cells (12,60,61). This stimulation will lead to the proliferation of ECL cells, which can lead to ECL cell hyperplasia and eventually ECL cell carcinoid tumors. HDC also acts as an intermediary response during this time to help replenish the histamine levels (12). Generally HDC can be found in high levels in gastric ECL, and in gastric adenocarcinoma it is regulated by gastrin via a protein kinase C dependent pathway (12). It has been shown that the HDC enzyme can be found in the stomach, and that its expression can help aid in stomach growth and development (12). The same experiment also proved that HDC expression is increased in gastric diseases such as hypergastrinemia (12). A recent study has shown that HDC positive cells are found in the gastric mucosa of normal gastric tissue (54). The ability to decrease histamine levels through the inhibition of histamine receptors may prove to be an effective method for blocking the growth of cancer cells in the stomach (1,55). When H2HR is stimulated by histamine in gastric carcinomas it has downstream effects that lead to the release of cAMP (58). The release of cAMP in high concentrations promotes the growth of gastric adenocarcinoma (62). Experiments have proven that the inhibition of H2HR by certain H2HR antagonists increases apoptosis in cancer cells (55). H4HR binding of its ligands leads to protective effects in experimental models of gastric mucosal damage, so this seems as if H4HR binding of histamine could lead to pro-cancer responses (1). One study has verified the fact that tumor-bearing animals exhibit increases in HDC mRNA and histamine concentration in the stomachs oxyntic mucosa (61). Although its been confirmed that the growth of gastric cancer develops through cAMP activation, which is mediated through the binding of histamine to its receptors, the exact pathobiology of gastric cancer is far from known and still requires extensive research (57).
Pancreatic cancer
Pancreatic carcinoma is a destructive form of cancer with a poor prognosis, being the fourth leading cause of cancer with an overall survival rate of five years (63-65). The causes of pancreatic cancer include the activation of oncogenes, inactivation of tumor suppressor genes, the deregulation of specific signaling pathways, hereditary pancreatitis, and tobacco smoking (63,66,67). In a cell line derived from human ductal pancreatic carcinoma it was shown that clonogenic growth was inhibited by histamine levels higher than 1 µmol•L-1 through H1HR and H2HR, but was stimulated by nanomolar doses of histamine (1,44,68). Anti-proliferation is induced by H2HR through G0/G1 phase arrest, decrease in phosphoactivated ERK1/ERK2, increase in phosphoactivated P38 expression, and modifications of the Bcl-2 family proteins (1,69). The H1HR receptor has also been shown to play a role in clonogenic growth inhibition (44). Partial cell differentiation has been associated with this growth inhibition, but the role of apoptosis is not significant in this anti-proliferative action (1,44,70). Recent studies have proven that H3HR and H4HR have a role in pancreatic carcinoma cell growth as well (1,24,44). Data suggests that H3HR increases proliferation through regulation of the cell cycle in normal and tumoral epithelial tissues (44,50,71). On the other hand, stimulation of H4HR in pancreatic cancer has proven to have a proliferative effect on tumoral cell growth (44,72). Notably, HDC over expression has been seen in neoplastic pancreatic cancers (10,14,42). One study has indicated that a majority of pancreatic endocrine tumors express HDC as well (10).
Colorectal cancer
Colorectal cancer (CRC) is one of the leading and most devastating forms of cancer-related deaths worldwide (1,73). In 2005, there were one million diagnosed cases of colorectal cancer over a 3-year diagnoses period (53). The development of colonic adenomas and carcinomas is caused by genetic and molecular alterations that can be induced by external and environmental factors, inflammatory bowel diseases, obesity, and alcohol consumption (74). When studying human colon tumors a high concentration of histamine was detected in the tumors as opposed to the surrounding tissues (1). CRC tumors contain abundant amounts of T-regulatory cells (Treg) and mast cells (75). In these tumors Treg shuts down IL10 and starts to express IL17; which promotes the expansion and degranulation of mast cells in the area, causing the release of histamine and other chemicals (75). It has also been shown that the absence or down regulation of HDC, through inhibition of mature myeloid cells or hypermethylation of the HDC promoter, has been experimentally proven to yield a higher rate of colon carcinogenesis (13). Correspondingly, patients with colonic adenomas have been shown to have down regulation of the amount of histamine catabolism in their colonic mucosa (76). Mice that contain HDC deficient immature myeloid cells (IMC’s) promoted the growth of tumor allografts; consequently, IMC’s that acquire exogenous histamine are differentiated and can suppress their ability to grow tumor allografts (13). These experiments demonstrated the vital role that histamine and HDC expression play myeloid cell differentiation, and the role of IMC’s in the development of early cancer (13). Figure 1 shows the number of colonic tumor numbers and demonstrates that, after loss of HDC, there is a significant increase in the number of both small and large colon tumors (Reprinted with permission from Yang et al. Nature Medicine 2011;17:87-95). Aside from lack of HDC, CRC tissues generally show abnormalities in H4HR expression (46). The over expression of H4HR combined with exposure to histamine has proven to cause growth arrest in CRC, and H4HR stimulation promotes CRC cellular apoptosis (46). Neoplastic samples contain decreased levels of H4HR compared to the amount detected in normal colon tissue samples, leading to the hypothesis that H4HR is involved in colon carcinogenesis (1). Aside from HRH4, it has also been experimentally verified that inhibition of H2HR through binding of certain antagonists’ increases the survival of patients with CRC (55,77-79). In some cases, this binding of H2HR to its antagonist seems to inhibit cell proliferation and will induce apoptosis in vitro (55,80).
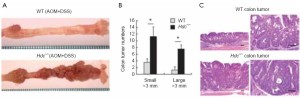
Hepatocellular carcinoma
Hepatocellular carcinoma (HCC) is one of the most common malignancies in the world with 598,000 deaths over a three-year diagnosis (53,81). Possible factors that give rise to the development of HCC are oxidative stress, chronic inflammation, hepatitis, alcohol abuse, and liver cirrhosis (81). In an experiment with two different HCC cell lines it was shown that stimulation via histamine could induce varying effects on the cancer cells by decreasing the amount of growth in one cell line but increasing the growth in the other (43,82). In the inhibited cell line it was shown that histamine controlled this effect through the down regulation of β-catenin, COX-2, and survivin expression in the cells (43). This down regulation worked in conjunction with the activation of caspase-3 and PARP cleavage to result in apoptotic responses in the cell line (43). This decrease in cellular proliferation and increase in apoptosis is possibly regulated by β-catenin, COX-2, and survivin through the activation of H1HR (43). In contrast, the other HCC cell line proliferated through histamine-induced up regulation of β-catenin and survivin expression (43). It is also possible that this regulation of β-catenin with survivin can act to increase cell survival through the activation of H2HR (43). In other hepatoma cell lines it has been demonstrated that histamine binding to H2HR can inhibit IL-6 binding to the cancer cells, which leads to less IL-6 expression and inhibition of cancer cell growth (83). Other studies have shown how H2HR antagonists can inhibit EGF-induced cellular proliferation and migration in HCC cell lines by decreasing cAMP concentration, and it can reduce liver metastasis by activating selectins in liver sinusoids (82,84,85). Aside from H2HR expression, intrahepatic and extrahepatic cell lines express H3HR as well (47,86). Experimentation has proven that H3HR agonist can decrease tumor growth and expression of VEGF and its receptors in vivo (86). Another hepatoma cell line showed that the binding of histamine to H3HR lead to antiproliferative effects, whereas H3HR antagonists increased cellular proliferation (47). This is evidence for an auto inhibitory H3HR in some hepatoma cell lines (47). The same hepatoma cell line showed an increase in HDC expression along with an increase in histamine synthesis (47). There was also a submicromolar extracellular histamine concentration along with a low intracellular histamine concentration indicating that the histamine is completely secreted from these hepatoma cells into the external medium (47,87). From this study we can conclude that histamine secreted from these hepatoma cells along with exogenous histamine can down regulate the proliferation of tumor-derived cell lines through its interaction with the auto inhibitory H3HR (47).
Apart from histamine, mast cells can have different roles in HCC depending on tumor cell features (43). Compared to normal cell lines, liver carcinoma shows an accumulation of mast cells demonstrating its role in tumor immunology (43,88). Mast cell concentration has proven to increase during carcinogenesis in HCC signifying its role in liver fibrosis and tumor immunology (88). Little is known about the direct relationship between mast cells and liver tumor growth, but there is evidence that tumor-associated mast cell accumulation can promote tumor growth and metastasis in certain conditions (43,89).
Hepatobiliary growth
Our last section will discuss the role of histamine and histamine receptors on cholangiocarcinoma regulation, but first we will give a brief background on biliary growth, as it is the growth of the biliary tree that can lead to cholangiocarcinoma. When bile is secreted from the liver (at the bile canaliculus) it travels down a pathway known as the biliary tree (90-92). The biliary tree is a three-dimensional structure comprised of different sized ducts, recognized as small and large ducts, which are lined by epithelial cells called cholangiocytes, also known as small and large cholangiocytes (90-92). Cholangiocytes are cuboidal groups of heterogeneous cells that branch from either the small or large bile ducts of the biliary tree (90-94). Cholangiocytes function to modify the biliary tree, release bile from the liver, and transport bile acids (93-98). Hormones that exert their effects via the cAMP pathway regulate the secretion of bile from cholangiocytes, and generally this function is used during pathogenesis of cholestatic liver diseases such as primary biliary cirrhosis and primary sclerosing cholangitis (93,99). These cholangiocytes are typically dormant but will start to proliferate in response to specific stimuli such as neuroendocrine factors, gastrointestinal hormones, and autocrine or paracrine signaling mechanisms (93,100-103). The proliferation of cholangiocytes is vital for maintaining the equilibrium of the biliary tree and cholangiopathies are the result of the biliary tree causing a loss of proliferative responses and an increase in apoptosis of the cholangiocytes (104). For this reason the proliferation of cholangiocytes occurs when there is some kind of liver damage or disease; such as chronic cholestatic liver diseases, partial hepatectomy, and extrahepatic bile duct obstruction induced by bile duct ligation (BDL) (104-108). Cholangiocyte proliferation allows for the compensation for any loss of cholangiocytes due to apoptosis, slow the progression of injury, and restoration of bile mass (109,110). These restoring responses have different pathways and functions in small and large cholangiocytes that are mediated through the binding of histamine or other agonists to H1HR, H2HR, H3HR, and H4HR (20,48,105,111). The actions mediated by these histamine receptors are vital for the regulation of hyperplastic and neoplastic growth of the biliary epithelium, and helps to play a role in the initiation and progression of liver fibrosis (20,48,105,111). It has been shown that activation of H1HR and H2HR promote growth of cholangiocytes, while H3HR and H4HR inhibit growth (20,48,105,111,112). Specifically, experiments have demonstrated how the binding of agonists to H1HR leads to small cholangiocyte proliferation through the activation of an IP3/Ca2+ dependent CaMK/CREB pathway (111). The activation of H3HR leads to the inhibition of large cholangiocyte growth in BDL rats by downregulating cAMP dependent PKA/ERK1/2/Ets-like gene-1 pathway (105). Small cholangiocytes can proliferate in conditions typical of those seen during large cholangiocyte damage (111,113,114). Figure 2 (top panel) depicts the known signaling pathways of histamine and histamine receptors during normal and pathological conditions (Reprinted with permission from Francis et al. J Hepatol 2012;56:1204-6).
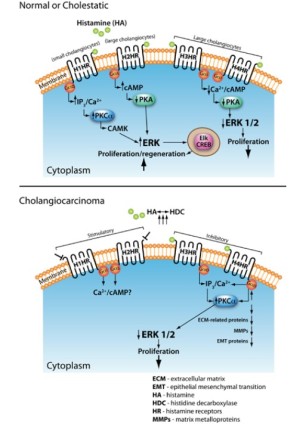
Cholangiocarcinoma
Cholangiocarcinoma (CCA) is a very rare malignancy seen in the tracts of the biliary tree and is caused by transformed epithelial cells (115-117). Often patients are diagnosed with CCA too late since it is hard to treat and difficult to distinguish from HCC (115,117,118). Even though CCA is not frequently diagnosed the rates of cases have risen worldwide over the past few decades (119,120). Forms of CCA can be either intrahepatic or extrahepatic and can form in any area of the biliary tree (117,119,120). CCA risk factors include biliary tract inflammation, liver flukes, hepatitis B and C, diabetes, liver cirrhosis, and other chronic liver diseases (121,122). We have recently shown that long-term treatment of CCA with histamine increases VEGF expression and proliferation (48,82). This proves the trophic effects of histamine on CCA, but the binding of histamine to its receptors can have different effects on proliferation (48,82). In the study by Francis et al. expression of H1-H4HR was found in numerous CCA cell lines, with an up regulation of H3HR. This study also showed that binding of H1HR to its antagonists leads to a decrease in CCA growth and an increase in apoptosis in vitro, whereas binding of H2HR to its antagonist has no effect on CCA cell lines (48,82). H3HR binding to its agonist have shown to activate the PKCα-dependent pathway, leading to inhibition of CCA growth in vitro and in vivo (86). This study demonstrates that H3HR signals via Gαο and induces an increase in intracellular IP3 but not cAMP signaling (86). The antiproliferative effects induced by H3HR also decreased VEGFA/C and VEGF-R2/3 expression, demonstrating that H3HR is a possible inhibitor of angiogenesis. This antiproliferative effect of H3HR works in CCA growth but has no effect on normal cholangiocytes (86). Decreases in CCA growth and invasion have proven to be mediated by some H3HR agonists and H4HR agonists through integrin modulated effects (48,82,86,112). Using an H4HR agonist, clobenpropit, Meng et al. human CCA metastasis potential and growth (both in vitro and in vivo) were inhibited and epithelial to mesenchymal transition was also blocked after treatment with clobenpropit (112). The effects of clobenpropit were mediated via a Ca2+-dependent mechanism and altered morphological development and invasion (112). Figure 2 (bottom panel) depicts the known signaling pathways of histamine and histamine receptors during cholangiocarcinoma growth (Reprinted with permission from Francis et al. J Hepatol 2012;56:1204-6). In a recent study, Francis et al. found that HDC is overexpressed in human cholangiocarcinoma when compared to normal tissues and cells. This study found that inhibition of HDC (both pharmacologically and genetically) inhibited the growth of cholangiocarcinoma in both cell cultures and in animal tumor models (48). Inhibition of HDC also significantly decreased VEGF expression in vitro and in vivo, but did not alter normal cholangiocyte growth (48) suggesting that this could be a favorable therapy for the treatment of human cholangiocarcinoma. We refer the reader to Figure 3 that demonstrates that after HDC knockdown in cholangiocarcinoma cells (Mz-HDC), xenograft tumor development was significantly inhibited when compared to the tumors containing normal levels of HDC (Mz-neg). Further, in tumor sections extracted from the animal model (that contain 99% cholangiocarcinoma cells), Francis et al. found that both proliferating cellular nuclear antigen and HDC expression were significantly decreased in the HDC knockdown transfected tumors (Mz-HDC) (Reprinted with permission from Francis et al. Gut, 2012;61:753-64.).
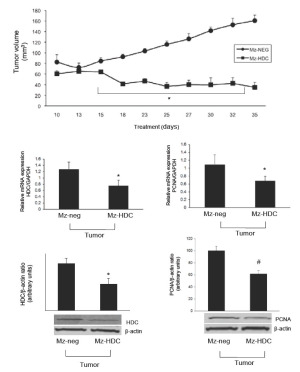
Concluding remarks
As demonstrated throughout this review the neurotransmitter, histamine, regulates numerous physiological responses within an organism. These various effects can be modulated through the binding of histamine to its receptors (H1-H4HR). Dependent on cell features and the microenvironment this binding can either be beneficial or detrimental to the cell in terms of growth. Histamine within cancerous cells can have varying responses such as increases or decreases in proliferation and angiogenesis. These different responses and their pathways require further investigation that could be used to help better describe their effects in certain malignancies. Since histamine has four receptors through which it can exert numerous reactions it is essential that future studies on the histamine receptors agonists and antagonists be performed. Tapping into the resourcefulness of these agonists and antagonists can be beneficial in terms of therapeutics. Understanding the specifics of different cancers, such as certain proteins expressed, chemicals that can bind to receptors on the cancerous cells, and different pathways utilized, can open up a multitude of options for types of cancer screenings, anti-cancer drugs, therapeutics and cancer prevention.
Acknowledgements
Portions of this work was partly supported by the NIH grants DK58411 and DK76898 and by the Scott & White Nicholas C. Hightower Endowed Chair of Gastroenterology (G. Alpini).
Disclosure: The authors declare no conflict of interest.
References
- Coruzzi G, Adami M, Pozzoli C. Role of histamine H4 receptors in the gastrointestinal tract. Front Biosci (Schol Ed) 2012;4:226-39.
- de Esch IJ, Thurmond RL, Jongejan A, et al. The histamine H4 receptor as a new therapeutic target for inflammation. Trends Pharmacol Sci 2005;26:462-9.
- Hegyesi H, Darvas Z, László V, et al. Retinoic acid enhances histamine content and H1 receptor expression in human neuroblastoma cell line Paju. Anticancer Res 2004;24:1657-63.
- Hill SJ, Ganellin CR, Timmerman H, et al. International Union of Pharmacology. XIII. Classification of histamine receptors. Pharmacol Rev 1997;49:253-78.
- Kahlson G, Rosengren E. New approaches to the physiology of histamine. Physiol Rev 1968;48:155-96.
- Zimmermann AS, Burhenne H, Kaever V, et al. Systematic analysis of histamine and N-methylhistamine concentrations in organs from two common laboratory mouse strains: C57Bl/6 and Balb/c. Inflamm Res 2011;60:1153-9.
- Medina VA, Brenzoni PG, Lamas DJ, et al. Role of histamine H4 receptor in breast cancer cell proliferation. Front Biosci (Elite Ed) 2011;3:1042-60.
- Ebo DG, Bridts CH, Mertens CH, et al. Analyzing histamine release by flow cytometry (HistaFlow): a novel instrument to study the degranulation patterns of basophils. J Immunol Methods 2012;375:30-8.
- Li Z, Liu J, Tang F, et al. Expression of non-mast cell histidine decarboxylase in tumor-associated microvessels in human esophageal squamous cell carcinomas. APMIS 2008;116:1034-42.
- Tanimoto A, Matsuki Y, Tomita T, et al. Histidine decarboxylase expression in pancreatic endocrine cells and related tumors. Pathol Int 2004;54:408-12.
- Yatsunami K, Fukui T, Ichikawa A. Molecular biology of L-histidine decarboxylase. Yakugaku Zasshi 1994;114:803-22.
- Höcker M, Zhang Z, Koh TJ, et al. The regulation of histidine decarboxylase gene expression. Yale J Biol Med 1996;69:21-33.
- Yang XD, Ai W, Asfaha S, et al. Histamine deficiency promotes inflammation-associated carcinogenesis through reduced myeloid maturation and accumulation of CD11b+Ly6G+ immature myeloid cells. Nat Med 2011;17:87-95.
- Medina VA, Rivera ES. Histamine receptors and cancer pharmacology. Br J Pharmacol 2010;161:755-67.
- Huszti Z, Magyar K, Keleti J. Possible regulation of hypothalamus and lung histidine decarboxylase activity by cAMP-dependent protein kinase. Eur J Biochem 1991;197:191-6.
- Savany A, Cronenberger L. Relationship between the multiple forms of rat gastric histidine decarboxylase: effects of conditions favouring phosphorylation and dephosphorylation. Biochem Int 1990;20:363-74.
- Rangachari PK. Histamine: mercurial messenger in the gut. Am J Physiol 1992;262:G1-13.
- Blaya B, Nicolau-Galmés F, Jangi SM, et al. Histamine and histamine receptor antagonists in cancer biology. Inflamm Allergy Drug Targets 2010;9:146-57.
- Falus A, Pós Z, Darvas Z. Histamine in normal and malignant cell proliferation. Adv Exp Med Biol 2011;709:109-23.
- Francis HL, Demorrow S, Franchitto A, et al. Histamine stimulates the proliferation of small and large cholangiocytes by activation of both IP3/Ca2+ and cAMP-dependent signaling mechanisms. Lab Invest 2012;92:282-94.
- Iwata K, Luo J, Penn RB, et al. Bimodal regulation of the human H1 histamine receptor by G protein-coupled receptor kinase 2. J Biol Chem 2005;280:2197-204.
- Hill SJ. Distribution, properties, and functional characteristics of three classes of histamine receptor. Pharmacol Rev 1990;42:45-83.
- Bryce PJ, Mathias CB, Harrison KL, et al. The H1 histamine receptor regulates allergic lung responses. J Clin Invest 2006;116:1624-32.
- Marshall JS, Jawdat DM. Mast cells in innate immunity. J Allergy Clin Immunol 2004;114:21-7.
- Svensjö E, Grega GJ. Evidence for endothelial cell-mediated regulation of macromolecular permeability by postcapillary venules. Fed Proc 1986;45:89-95.
- Van Amsterdam RG, Meurs H, Brouwer F, et al. Role of phosphoinositide metabolism in functional antagonism of airway smooth muscle contraction by beta-adrenoceptor agonists. Eur J Pharmacol 1989;172:175-83.
- Gespach C, Bouhours D, Bouhours JF, et al. Histamine interaction on surface recognition sites of H2-type in parietal and non-parietal cells isolated from the guinea pig stomach. FEBS Lett 1982;149:85-90.
- Kanof PD, Greengard P. Pharmacological properties of histamine-sensitive adenylate cyclase from guinea pig cardiac ventricular muscle. Mol Pharmacol 1979;15:445-61.
- Falus A, Merétey K. Histamine: an early messenger in inflammatory and immune reactions. Immunol Today 1992;13:154-6.
- Leino L, Lilius EM. Histamine receptors on leukocytes are expressed differently in vitro and ex vivo. Int Arch Allergy Appl Immunol 1990;91:30-5.
- Arrang JM, Devaux B, Chodkiewicz JP, et al. H3-receptors control histamine release in human brain. J Neurochem 1988;51:105-8.
- Cherifi Y, Pigeon C, Le Romancer M, et al. Purification of a histamine H3 receptor negatively coupled to phosphoinositide turnover in the human gastric cell line HGT1. J Biol Chem 1992;267:25315-20.
- Schlicker E, Schunack W, Göthert M. Histamine H3 receptor-mediated inhibition of noradrenaline release in pig retina discs. Naunyn Schmiedebergs Arch Pharmacol 1990;342:497-501.
- Grandi D, Shenton FC, Chazot PL, et al. Immunolocalization of histamine H3 receptors on endocrine cells in the rat gastrointestinal tract. Histol Histopathol 2008;23:789-98.
- Liu C, Ma X, Jiang X, et al. Cloning and pharmacological characterization of a fourth histamine receptor [H(4)] expressed in bone marrow. Mol Pharmacol 2001;59:420-6.
- Nijmeijer S, de Graaf C, Leurs R, et al. Molecular pharmacology of histamine H4 receptors. Front Biosci 2012;17:2089-106.
- Oda T, Morikawa N, Saito Y, et al. Molecular cloning and characterization of a novel type of histamine receptor preferentially expressed in leukocytes. J Biol Chem 2000;275:36781-6.
- Zhu Y, Michalovich D, Wu H, et al. Cloning, expression, and pharmacological characterization of a novel human histamine receptor. Mol Pharmacol 2001;59:434-41.
- Dunford PJ, O’Donnell N, Riley JP, et al. The histamine H4 receptor mediates allergic airway inflammation by regulating the activation of CD4+ T cells. J Immunol 2006;176:7062-70.
- Hofstra CL, Desai PJ, Thurmond RL, et al. Histamine H4 receptor mediates chemotaxis and calcium mobilization of mast cells. J Pharmacol Exp Ther 2003;305:1212-21.
- Darvas Z, Sakurai E, Schwelberger HG, et al. Autonomous histamine metabolism in human melanoma cells. Melanoma Res 2003;13:239-46.
- Rivera ES, Cricco GP, Engel NI, et al. Histamine as an autocrine growth factor: an unusual role for a widespread mediator. Semin Cancer Biol 2000;10:15-23.
- Lampiasi N, Azzolina A, Montalto G, et al. Histamine and spontaneously released mast cell granules affect the cell growth of human hepatocellular carcinoma cells. Exp Mol Med 2007;39:284-94.
- Cricco GP, Mohamad NA, Sambuco LA, et al. Histamine regulates pancreatic carcinoma cell growth through H3 and H4 receptors. Inflamm Res 2008;57:S23-4.
- Dyduch G, Kaczmarczyk K, Okoń K. Mast cells and cancer: enemies or allies? Pol J Pathol 2012;63:1-7.
- Fang Z, Yao W, Xiong Y, et al. Attenuated expression of HRH4 in colorectal carcinomas: a potential influence on tumor growth and progression. BMC Cancer 2011;195:1-11.
- Davenas E, Rouleau A, Morisset S, et al. Autoregulation of McA-RH7777 hepatoma cell proliferation by histamine H3 receptors. J Pharmacol Exp Ther 2008;326:406-13.
- Francis H, DeMorrow S, Venter J, et al. Inhibition of histidine decarboxylase ablates the autocrine tumorigenic effects of histamine in human cholangiocarcinoma. Gut 2012;61:753-64.
- Hegyesi H, Horváth B, Pállinger E, et al. Histamine elevates the expression of Ets-1, a protooncogen in human melanoma cell lines through H2 receptor. FEBS Lett 2005;579:2475-9.
- Medina V, Cricco G, Nuñez M, et al. Histamine-mediated signaling processes in human malignant mammary cells. Cancer Biol Ther 2006;5:1462-71.
- Medina V, Croci M, Crescenti E, et al. The role of histamine in human mammary carcinogenesis: H3 and H4 receptors as potential therapeutic targets for breast cancer treatment. Cancer Biol Ther 2008;7:28-35.
- Corso G, Seruca R, Roviello F. Gastric cancer carcinogenesis and tumor progression. Ann Ital Chir 2012;83:172-6.
- Parkin DM, Bray F, Ferlay J, et al. Global cancer statistics, 2002. CA Cancer J Clin 2005;55:74-108.
- Bakkelund KE, Nordrum IS, Fossmark R, et al. Gastric carcinomas localized to the cardia. Gastroenterol Res Pract 2012;2012:457831.
- Jiang CG, Liu FR, Yu M, et al. Cimetidine induces apoptosis in gastric cancer cells in vitro and inhibits tumor growth in vivo. Oncol Rep 2010;23:693-700.
- Prinz C, Schwendy S, Voland P. H pylori and gastric cancer: shifting the global burden. World J Gastroenterol 2006;12:5458-64.
- Micev M, Cosić-Micev M. Pathology and pathobiology of the gastric carcinoma. Acta Chir Iugosl 2011;58:39-52.
- Emami S, Gespach C, Forgue-Lafitte ME, et al. Histamine and VIP interactions with receptor-cyclic AMP systems in the human gastric cancer cell line HGT-1. Life Sci 1983;33:415-23.
- Håkanson R, Böttcher G, Ekblad E, et al. Histamine in endocrine cells in the stomach. A survey of several species using a panel of histamine antibodies. Histochemistry 1986;86:5-17.
- Hakanson R, Sundler F. Trophic effects of gastrin. Scand J Gastroenterol 1991;26:130-6.
- Kölby L, Wängberg B, Ahlman H, et al. Altered influence of CCK-B/gastrin receptors on HDC expression in ECL cells after neoplastic transformation. Regul Pept 1999;85:115-23.
- Kong WQ, Bai R, Liu T, et al. MicroRNA-182 targets cAMP-responsive element-binding protein 1 and suppresses cell growth in human gastric adenocarcinoma. FEBS J 2012;279:1252-60.
- Iovanna J, Mallmann MC, Gonçalves A, et al. Current knowledge on pancreatic cancer. Front Oncol 2012;2:6.
- Roberts NJ, Jiao Y, Yu J, et al. ATM mutations in patients with hereditary pancreatic cancer. Cancer Discov 2012;2:41-6.
- Zakharova OP, Karmazanovsky GG, Egorov VI. Pancreatic adenocarcinoma: Outstanding problems. World J Gastrointest Surg 2012;4:104-13.
- Nitsche C, Simon P, Weiss FU, et al. Environmental risk factors for chronic pancreatitis and pancreatic cancer. Dig Dis 2011;29:235-42.
- Sarkar FH, Banerjee S, Li Y. Pancreatic cancer: pathogenesis, prevention and treatment. Toxicol Appl Pharmacol 2007;224:326-36.
- Cricco G, Martin G, Labombarda F, et al. Human pancreatic carcinoma cell line Panc-I and the role of histamine in growth regulation. Inflamm Res 2000;49:S68-9.
- Cricco G, Martín G, Medina V, et al. Histamine inhibits cell proliferation and modulates the expression of Bcl-2 family proteins via the H2 receptor in human pancreatic cancer cells. Anticancer Res 2006;26:4443-50.
- Martín G, Cricco G, Darvas Z, et al. Histamine inhibits proliferation of a pancreatic carcinoma cell line without inducing apoptosis significantly. Inflamm Res 2002;51:S67-8.
- Grandi D, Schunack W, Morini G. Epithelial cell proliferation is promoted by the histamine H(3) receptor agonist (R)-alpha-methylhistamine throughout the rat gastrointestinal tract. Eur J Pharmacol 2006;538:141-7.
- Cianchi F, Cortesini C, Schiavone N, et al. The role of cyclooxygenase-2 in mediating the effects of histamine on cell proliferation and vascular endothelial growth factor production in colorectal cancer. Clin Cancer Res 2005;11:6807-15.
- Rawson JB, Bapat B. Epigenetic biomarkers in colorectal cancer diagnostics. Expert Rev Mol Diagn 2012;12:499-509.
- Hasan N, Pollack A, Cho I. Infectious causes of colorectal cancer. Infect Dis Clin North Am 2010;24:1019-39,x.
- Blatner NR, Bonertz A, Beckhove P, et al. In colorectal cancer mast cells contribute to systemic regulatory T-cell dysfunction. Proc Natl Acad Sci USA 2010;107:6430-5.
- García-Faroldi G, Sánchez-Jiménez F, Fajardo I. The polyamine and histamine metabolic interplay in cancer and chronic inflammation. Curr Opin Clin Nutr Metab Care 2009;12:59-65.
- Adams WJ, Morris DL. Short-course cimetidine and survival with colorectal cancer. Lancet 1994;344:1768-9.
- Kelly MD, King J, Cherian M, et al. Randomized trial of preoperative cimetidine in patients with colorectal carcinoma with quantitative assessment of tumor-associated lymphocytes. Cancer 1999;85:1658-63.
- Morris DL, Adams WJ. Cimetidine and colorectal cancer--old drug, new use? Nat Med 1995;1:1243-4.
- Rajendra S, Mulcahy H, Patchett S, et al. The effect of H2 antagonists on proliferation and apoptosis in human colorectal cancer cell lines. Dig Dis Sci 2004;49:1634-40.
- Wang XW, Hussain SP, Huo TI, et al. Molecular pathogenesis of human hepatocellular carcinoma. Toxicology 2002;181-182:43-7.
- Onori P, Gaudio E, Franchitto A, et al. Histamine regulation of hyperplastic and neoplastic cell growth in cholangiocytes. World J Gastrointest Pathophysiol 2010;1:38-49.
- Merétey K, Falus A, Taga T, et al. Histamine influences the expression of the interleukin-6 receptor on human lymphoid, monocytoid and hepatoma cell lines. Agents Actions 1991;33:189-91.
- Fujikawa T, Shiraha H, Nakanishi Y, et al. Cimetidine inhibits epidermal growth factor-induced cell signaling. J Gastroenterol Hepatol 2007;22:436-43.
- Lefranc F, Yeaton P, Brotchi J, et al. Cimetidine, an unexpected anti-tumor agent, and its potential for the treatment of glioblastoma (review). Int J Oncol 2006;28:1021-30.
- Francis H, Onori P, Gaudio E, et al. H3 histamine receptor-mediated activation of protein kinase Calpha inhibits the growth of cholangiocarcinoma in vitro and in vivo. Mol Cancer Res 2009;7:1704-13.
- Brandes LJ, Queen GM, LaBella FS. Displacement of histamine from liver cells and cell components by ligands for cytochromes P450. J Cell Biochem 2002;85:820-4.
- Terada T, Matsunaga Y. Increased mast cells in hepatocellular carcinoma and intrahepatic cholangiocarcinoma. J Hepatol 2000;33:961-6.
- Tóth-Jakatics R, Jimi S, Takebayashi S, et al. Cutaneous malignant melanoma: correlation between neovascularization and peritumor accumulation of mast cells overexpressing vascular endothelial growth factor. Hum Pathol 2000;31:955-60.
- Alpini G, Glaser S, Robertson W, et al. Large but not small intrahepatic bile ducts are involved in secretin-regulated ductal bile secretion. Am J Physiol 1997;272:G1064-74.
- Alpini G, Roberts S, Kuntz SM, et al. Morphological, molecular, and functional heterogeneity of cholangiocytes from normal rat liver. Gastroenterology 1996;110:1636-43.
- Kanno N, LeSage G, Glaser S, et al. Functional heterogeneity of the intrahepatic biliary epithelium. Hepatology 2000;31:555-61.
- Alpini G, McGill JM, Larusso NF. The pathobiology of biliary epithelia. Hepatology 2002;35:1256-68.
- Kanno N, LeSage G, Glaser S, et al. Regulation of cholangiocyte bicarbonate secretion. Am J Physiol Gastrointest Liver Physiol 2001;281:G612-25.
- Alpini G, Glaser SS, Ueno Y, et al. Bile acid feeding induces cholangiocyte proliferation and secretion: evidence for bile acid-regulated ductal secretion. Gastroenterology 1999;116:179-86.
- Alpini G, Kanno N, Phinizy JL, et al. Tauroursodeoxycholate inhibits human cholangiocarcinoma growth via Ca2+-, PKC-, and MAPK-dependent pathways. Am J Physiol Gastrointest Liver Physiol 2004;286:G973-82.
- Alpini G, Lenzi R, Sarkozi L, et al. Biliary physiology in rats with bile ductular cell hyperplasia. Evidence for a secretory function of proliferated bile ductules. J Clin Invest 1988;81:569-78.
- Xia X, Francis H, Glaser S, et al. Bile acid interactions with cholangiocytes. World J Gastroenterol 2006;12:3553-63.
- Strazzabosco M. Transport systems in cholangiocytes: their role in bile formation and cholestasis. Yale J Biol Med 1997;70:427-34.
- Alvaro D, Mancino MG, Glaser S, et al. Proliferating cholangiocytes: a neuroendocrine compartment in the diseased liver. Gastroenterology 2007;132:415-31.
- Glaser SS, Gaudio E, Miller T, et al. Cholangiocyte proliferation and liver fibrosis. Expert Rev Mol Med 2009;11:e7.
- Glaser SS, Ueno Y, DeMorrow S, et al. Knockout of alpha-calcitonin gene-related peptide reduces cholangiocyte proliferation in bile duct ligated mice. Lab Invest 2007;87:914-26.
- Polimeno L, Azzarone A, Zeng QH, et al. Cell proliferation and oncogene expression after bile duct ligation in the rat: evidence of a specific growth effect on bile duct cells. Hepatology 1995;21:1070-8.
- Marzioni M, Glaser S, Francis H, et al. Autocrine/paracrine regulation of the growth of the biliary tree by the neuroendocrine hormone serotonin. Gastroenterology 2005;128:121-37.
- Francis H, Franchitto A, Ueno Y, et al. H3 histamine receptor agonist inhibits biliary growth of BDL rats by downregulation of the cAMP-dependent PKA/ERK1/2/ELK-1 pathway. Lab Invest 2007;87:473-87.
- Francis H, LeSage G, DeMorrow S, et al. The alpha2-adrenergic receptor agonist UK 14,304 inhibits secretin-stimulated ductal secretion by downregulation of the cAMP system in bile duct-ligated rats. Am J Physiol Cell Physiol 2007;293:C1252-62.
- Lesage G, Glaser SS, Gubba S, et al. Regrowth of the rat biliary tree after 70% partial hepatectomy is coupled to increased secretin-induced ductal secretion. Gastroenterology 1996;111:1633-44.
- Mancinelli R, Onori P, Gaudio E, et al. Taurocholate feeding to bile duct ligated rats prevents caffeic acid-induced bile duct damage by changes in cholangiocyte VEGF expression. Exp Biol Med (Maywood) 2009;234:462-74.
- Jensen K, Marzioni M, Munshi K, et al. Autocrine regulation of biliary pathology by activated cholangiocytes. Am J Physiol Gastrointest Liver Physiol 2012;302:G473-83.
- Munshi MK, Priester S, Gaudio E, et al. Regulation of biliary proliferation by neuroendocrine factors: implications for the pathogenesis of cholestatic liver diseases. Am J Pathol 2011;178:472-84.
- Francis H, Glaser S, Demorrow S, et al. Small mouse cholangiocytes proliferate in response to H1 histamine receptor stimulation by activation of the IP3/CaMK I/CREB pathway. Am J Physiol Cell Physiol 2008;295:C499-513.
- Meng F, Han Y, Staloch D, et al. The H4 histamine receptor agonist, clobenpropit, suppresses human cholangiocarcinoma progression by disruption of epithelial mesenchymal transition and tumor metastasis. Hepatology 2011;54:1718-28.
- Alpini G, Franchitto A, Demorrow S, et al. Activation of alpha(1) -adrenergic receptors stimulate the growth of small mouse cholangiocytes via calcium-dependent activation of nuclear factor of activated T cells 2 and specificity protein 1. Hepatology 2011;53:628-39.
- Mancinelli R, Franchitto A, Gaudio E, et al. After damage of large bile ducts by gamma-aminobutyric acid, small ducts replenish the biliary tree by amplification of calcium-dependent signaling and de novo acquisition of large cholangiocyte phenotypes. Am J Pathol 2010;176:1790-800.
- Landis SH, Murray T, Bolden S, et al. Cancer statistics, 1998. CA Cancer J Clin 1998;48:6-29.
- Parkin DM, Ohshima H, Srivatanakul P, et al. Cholangiocarcinoma: epidemiology, mechanisms of carcinogenesis and prevention. Cancer Epidemiol Biomarkers Prev 1993;2:537-44.
- Patel T. Worldwide trends in mortality from biliary tract malignancies. BMC Cancer 2002;2:10.
- Sampatanukul P, Leong AS, Kosolbhand P, et al. Proliferating ductules are a diagnostic discriminator for intrahepatic cholangiocarcinoma in FNA biopsies. Diagn Cytopathol 2000;22:359-63.
- Blechacz B, Gores GJ. Cholangiocarcinoma: advances in pathogenesis, diagnosis, and treatment. Hepatology 2008;48:308-21.
- Blechacz BR, Gores GJ. Cholangiocarcinoma. Clin Liver Dis 2008;12:131-50, ix.
- Palmer WC, Patel T. Are common factors involved in the pathogenesis of primary liver cancers? A meta-analysis of risk factors for intrahepatic cholangiocarcinoma. J Hepatol 2012;57:69-76.
- Tischoff I, Tannapfel A. Hepatocellular carcinoma and cholangiocarcinoma--different prognosis, pathogenesis and therapy. Zentralbl Chir 2007;132:300-5.