Targeted therapy of obesity-associated colon cancer
Abstract
Obesity is increasing worldwide and has become a major health problem. It not only increases the incidence of colon cancer but also leads to poorer prognosis. The mechanisms could be the activation of multiple signaling pathways including PI3K/ Akt, MAPK and STAT3 that are induced by increased levels of cancer risk factors in obesity. Activation of these signaling pathways can cause drug resistance to chemotherapeutic agents, leading to poorer treatment outcome. Thus, inhibition of these pathways may increase the therapeutic efficacy of obesity-associated colon cancer. Many small molecule inhibitors have been developed for selection. Inhibition of a single signaling molecule may be not sufficient to reverse the activation of signals in obesity. Dual inhibitors for PI3K and mTOR or dual inhibitors for Akt and MAPK may be more effective. Combinational use of inhibitors could also increase treatment efficacy.
Key words
Obesity-associated colon cancer; multiple signal pathways; targeted therapy; PI3K/Akt; MAPK; STAT3
Introduction
Obesity is defined as body mass index (BMI) 30 and is now recognised as a major health problem worldwide (1). Epidemiological studies showed that about one third of the population in developed countries is obese (2). Obesity is also increasing in developing countries. For example in China, obesity is increased from 2.6% in a national survey in 2002 to about 5% in a recent survey (3-5). There are more than 20% of obese populations in some cities in China. Although the percentage of obesity in the Chinese population is still lower than that in developed countries, the total number of obese people is high due to a total population of 1.3 billion in China. Obesity can cause many comorbidities including type 2 diabetes, hypertension, hyperlipidemia, heart disease and cancer. As obesity is difficult to be prevented and treated, its co-morbidities must be well studied so that they can be effectively prevented or treated. It is now clear that obesity can increase the incidence of many cancers including colon cancer and lead to poorer treatment outcome (6-10). This is associated with the altered multiple cancer risk factors in obesity including increased serum levels of insulin, insulin-like growth factor-1 (IGF-1), leptin, Interleukine-6 (IL-6), IL- 17, tumo necrosis factor-alpha (TNF-alpha) and decreased serum levels of adiponectin (7,11-13). These altered factors in turn result in the activation of multiple signaling pathways including phosphoinositide 3-kinase/protein kinase B (PI3K/Akt), mitogen-activated protein kinase (MAPK) and signal tranducer and activator of transcription 3 (STAT3) pathways (Figure 1) (14-17). Activation of these signaling pathways is important for the drug resistance to clinical therapy, leading to poorer outcome of treatment (17-19). Thus, inhibition of multiple signaling pathways could improve the treatment efficacy of obesityassociated cancer. The study of obesity-associated cancer is urgent because this disease can result in increased mortality (9,10).
Colorectal cancer is the third most common cancer in males and the second in females worldwide with estimated death number of 608,700 in 2008 (20). It is caused by both environmental factors such as dietary intake and genetic factors such as inherited or somatic gene mutations (21). These factors lead to the activation of several signaling pathways. Several gene mutations are shown to activate survival signaling pathways. Inactivating gene mutation of Adenomatous polyposis coli (APC) is most studied in colorectal cancer (21). It can result in the accumulation of beta-catenin in Wnt signaling pathway, which in turn increases transcription of many oncogenes. KRAS is activating mutated in about 40% of colon cancer which can activate both PI3K and MAPK pathways (22). PTEN inactivating mutation and PIK3CA activating mutation lead to the activation of the PI3K/Akt pathway (21). Oncogene CMYC is activated in 10% of colorectal cancer (23). The carcinogenesis of colon cancer starts from the early lesion of the formation of polyps and some of these polyps develop into adenocarcinoma dependent on the progressive accumulation of mutations in key regulatory genes (24). Inoperable colon cancer requires treatment by chemotherapy. The main chemotherapeutic agents for colon cancer are 5-fluorouracil (5-FU), oxaliplatin and irinotecan. 5-FU inhibits the enzyme thymidylate synthase to affect DNA replication. Oxaliplatin also interferes with DNA replication by covalently binding to DNA to form platinum- DNA adducts. Irrinotecan is semisynthesized from camptothecin and inhibits topoisomerase to prevent DNA from unwinding. The success of treatment will depend on the initial effectiveness of the drugs and reduced drug efficacy is a major problem. The initial response to these drugs is about 50%. The low responses are caused by many factors including increased drug metabolism and transportation out of target cells (25). Obesity is known to impact colon cancer by activation of multiple signaling pathways. As multiple signaling pathways are activated and play important roles in many obesity-associated colon cancers, inhibition of these signaling pathways could increase treatment efficacy (26). This review will summarise the evidence of poorer prognosis of obesity-associated colon cancer and propose treatment strategies for the inhibition of signaling pathways and several novel approaches to improve the treatment of obesity-associated colon cancer.
Obesity and poorer outcome of colon cancer treatment
Epidemiological evidence has shown that the outcome of obesityassociated cancer is much poorer than colon cancer without obesity. Calle demonstrated that obesity increased the mortality of many cancers including colon cancer (27). In an epidemiological study in 39 Asian- Pacific Cohorts, it was also found that obesity increased significantly the risk of mortality from colon cancer (28,29). The study showed that there were 4872 deaths among 401,215 patients within 3 years. The mortality risk is increased in colon cancer in obese patients compared with those with normal weight and the hazard ratio is 1.50 (28).
Laboratory studies have provided supportive evidence for the epidemiological studies. Several studies demonstrated that cancer risk factors, which are increased in obesity, have caused drug resistance and poorer prognosis of obesity-associated colon cancer (6). Addition of insulin into cultured colon cancer cell HT29 resulted in the drug resistance to chemotherapeutic agents oxaliplatin and 5FU (30,31). The drug resistance is mediated at least partially by the activation of the PI3K/Akt pathway. Leptin has been demonstrated to make colon cancer worse by increasing metastasis and drug resistance via multiple signaling pathways (32-37). In colon cancer stem cells, leptin activated both Akt and MAPK pathways (32). This not only increases cell proliferation but also intereferes with the treatment efficacy of 5-FU.
Inhibition of multiple signaling pathways as a strategy for the treatment of obesity-associated colon cancer
As activated multiple signaling pathways in obesity play critical roles in the treatment outcome of obesity-associated colon cancer, reversing these pathways to normal status could increase the treatment efficacy of the disease. Treatment of obesity may be able to make such reverse. However, there is no effective treatment for obesity at present. Therefore, small molecule signaling pathway inhibitors could be chosen for the treatment of obesity-associated colon cancer for targeted therapy (Figure 1, 2). The objectives are to choose the most effective nodes of these signaling pathways for the inhibition and avoid the side-effects as much as possible. Simultaneous inhibition of two or more nodes of these signaling pathways could also be chosen to increase effectiveness.
As colon cancer is a heterogenous disease, the detailed plan for targeted therapy should be worked out dependent on the activation status of signal pathways in an individual patient. The development status of obesity in an individual patient could be assessed for estimation of the activation of multiple signal pathways. Other mutations in an individual patient may also shape the signal pathways in the patient, making it different from others. The actual activities of these signal pathways may be determined for the detailed targeted therapy plan.
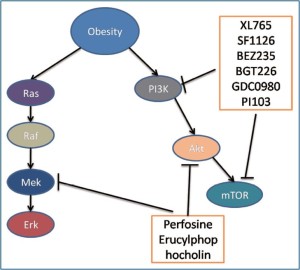
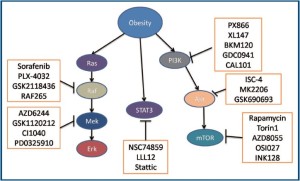
Inhibition of the PI3K/Akt pathway
The PI3K/Akt pathway plays a central role in cell proliferation, cell survival, cell growth, cell metabolism, cell motility, angiogenesis and genomic stability. It is activated by receptor tyrosine kinases such as epidermal growth factor receptor (EGFR), platelet derived growth factor receptor, insulin receptor, insulin-like growth factor-1 receptor and regulates a cascade of down-stream targets. Several excellent reviews are available for the details of the pathway (38,39). The pathway is activated in many cancers including colon cancer. Activation of the PI3K/Akt pathway in cancer has been associated with decreased drug efficacy in the treatment and inhibition of the pathway increases the sensitivity of cancer cells to cytotoxic agents (40). Many inhibitors have been developed for the pathway to target its major components, especially now the dual inhibitors targeting both PI3K and its downstream target–mammalian target of rapamycin (mTOR) are available. Inhibition of the pathway has been used for the treatment of colon cancer.
In obesity-associated colon cancer, it has been shown that the PI3K/ Akt pathway is activated and inhibition of the pathway by specific inhibitors or food restriction reduces the incidence of colon cancer (41-43). In an in vitro model, insulin which is increased in obesity was shown to increase drug resistance to 5-FU and oxaliplatin via the activation of the PI3K/Akt pathway. Inhibition of the pathway by Ly294002 restored the colon cancer cell sensitivity to these two chemotherapeutic agents (43). This may be further studied in animal models in order to be used in clinical trials. Therefore PI3K/Akt pathway inhibitors may be useful to improve the therapeutic effectiveness of chemotherapy for obesityassociated colon cancer. These signaling pathway inhibitors are listed in Table 1 and discussed in detail below.
Signaling molecules | PI3K | Akt | mTOR | PI3K/mTOR |
Inhibitors | PX-866, | ISC-4 | Rapamycin | XL765, |
XL147, | MK-2206 | Torin1 | SF1126, | |
BKM120, | GSK690693 | AZD-8055 | BEZ235, | |
GDC-0941, | OSI-027 | BGT226, | ||
CAL-101 | INK128 | GDC-0980 | ||
PI-103 |
PI3K inhibitors
There are three classes of PI3K but only class I is closely related with cancer (39). Class I PI3K consists of a regulatory unit p85 and a catalytic unit p110. There are 5 isoforms of p85 including p85 alpha, beta, p55 alpha, p55 gamma and p50 alpha. The isoforms of p110 contain p110 alpha, beta, gamma and delta. Several PI3K inhibitors are described below.
PX-866 (Oncothyreon, Seattle, WA, USA)
PX-866 is a pan-isoform PI3K inhibitor derived from wortmannin. It has been shown to inhibit PI3K in colon cancer HT29 cells with an IC50 of 0.1nM (44). In an HT29 xenograft colon cancer mouse model, it inhibited PI3K, as indicated by decreased phosphorylation of Akt (45). One phase I clinical trial in advanced solid tumors for the tolerance study with escalated dosage has completed (NCT00726583) and four phase I and II clinical trials are recruiting participants. The most noted side-effect of PX-866 is hyperglycemia which can be overcome by pioglitazone (46).
XL147 (Sonofi-aventis)
XL147 selectively inhibits class I PI3K isoforms by binding to the isoforms in an ATP-competitive manner. In a phase I clinical trial, XL147 decreased phosphorylation of Akt and its down-steam target S6 in several cancer cell lines, resulting in inhibition of cell growth (47). It was also demonstrated to be effective against tumo growth in the xenograft models of several cancers. In addition, XL147 has an additive effect with chemotherapeutic drugs in breast cancer (47). A problem in using XL147 was subsequent up-regulation of HER3 which attenuated the effect of XL147 (48). In a xenograft model, HER2 and HER3 antagonists were shown to have synergistic effects with XL147. The maximum tolerated dose (MTD) was identified to be 600mg. At this dose, PI3K was inhibited and prolonged stable diseases have been achieved. The side-effects of XL147 include skin rash, arterial thrombosis, transaminase elevation and hyperglycermia. At present, a phase II clinical trial is recruiting participants (NCT1013324).
BKM120 (Novartis)
BKM120 is a pan-PI3K inhibitor and has been testead in several cancers including melanoma, medulloblastoma and breast cancer (49-51). It was shown to inhibit the growth of melanoma cells and has asynergistic effect with rapamycin (49). In medulloblastoma, the inhibitor of a protein called G-protein Smoothened (Smo) can cause tumor regression in an animal model (50). However, activation of PI3K/Akt can lead to drug resistance to Smo inhibitors. BKM120 overcome the development of such drug resistance. In ER-positive breast cancers, BKM120 alone can lead to apoptosis under the condition that estrogen is deprived (51). Two clinical trials using BKM120 are currently recruiting (NCT01300962 and NCT01297491).
GDC-0941 (Genentech)
GDC-0941 is a potent inhibitor of the PI3K isoform p110 alpha and has high selectivity against class I PI3K (52). It has been tested in several cancers including breast ovarian, lung and prostate cancer (52-54). In breast cancer, ABT-737 can cause apoptosis but its effect is modest. GDC-0941 has been shown to have strong synergistic cytotoxicity with ABT-737 (54). In an MDA-MB-231 xenograft breast cancer model, their combinational use had also greater effects than one agent alone. Several clinical trials for GDC-0941 are recruiting (www.clinicaltrials.gov).
CAL-101 (Calistoga Phamaceuticals, Inc.)
CAL-101 is the PI3K inhibitor which has been tested in haematological malignancies (55-62). It is a selective inhibitor of p110 delta. Inhibition of the isoform by CAL-101 has been shown to decrease pAkt and its down-stream targets, and cause increased caspase cleavage and apoptosis in B-cell leukemia (60).
Akt inhibitors
Akt, a serine/threonine kinase, is the major mediator of the PI3K and used as an indicator for the activation of the pathway (39). There are three isoforms of Akt; Akt 1, Akt 2 and Akt 3 encoded by three distinct genes located in 14q32, 19q13 and 1q44 respectively (63). All of them include an N-terminal pleckstrin homology (PH) domain, a regulatory hydrophobic C-terminal domain and a central kinase domain. PH interacts with PIP3 and directs Akt to locate to the plasma membrane for phosphorylation by phosphoinositide-dependent kinase 1. The Akt inhibitors were developed recently.
ISI-4
ISC-4 is synthesized from natural product isothiocyanate (64). Isothiocyanate was found to have anti-cancer effect in screening against Akt3. It was further chemically modified to be isoselenoocyanates by increasing alkyl side chains and substituting sulfur with selenium to be more potent. ISC-4, which has four carbon alkyl side chains, was tested to be effective against colon cancer (65). In a mouse model with HT29 colon cancer cell xenografts, ISC-4 increased the effect iveness of 5-FU treatment.
MK-2206 (Merck)
MK-2206 is an allostetic inhibitor of all three Akt isoforms. Preclinical studies showed that it is effective on several cancers. A phase I clinical trial to test MK-2206 in solid cancers has now been conducted (66). In thirty three patients, the agent was given at the doses of 30, 60, 75 and 90 mg on alternative days and the MTD was identified to be 60 mg. At this dose, the phosphoryaltion of Akt at Ser473 was found to be decreased and the tumors were shrinked. The most noted side-effects were skin rash and stomatitis. MK-2206 has synergistic effects with anti-cancer agents erotinib, lapatinib, doxorubincin, camptothecin, gemcitabine, 5-FU, carboplatin and docetaxel (67).
mTOR inhibitors
mTOR, a serine-threonine kinase discovered in 1994, is a major mediator in the PI3K/Akt pathway (68-70). It is also activated by MAPK pathway. mTOR is a 2594 amino acid peptide with a molecular weight of 289 kDa and is included in two mTOR complexes. mTORC1 consists of mTOR, regulatory associated protein of mTOR, mammalian LST8/G protein -subunit like protein (mLST8GL), PRAS40 and DEPTOR while mTORC2 contains mTOR, GL, rapamycin-insensitive companion of mTOR (Rictor), and mammalian stress-activated protein kinase interacting protein 1 (71,72). Both complexies play important roles in colon cancer tumorigenesis and progression (73,74). Traditional inhibitor of mTOR rapamycin can only inhibit mTORC1. New developed mTOR inhibitors torin 1, AZD8055, INK128 and OSI can inhibit both mTORC1 and mTORC2.
Rapamycin (Wyeth)
Rapamycin, a macrolide antibiotic isolated from the soil bacterium Streptomyces hygroscopicus, is used clinically as an immunosuppressive agent in organ transplantation (75). It has been tested to be effective for colon cancer (73,74,76-78). Rapamycin can inhibit mTORC1 and reduce colon cancer cell migration and invasion (74). Rapamycin in combination with irinotecan greatly inhibited mTOR and its downstream target hypoxia-inducible factor 1 and resulted in greater reduction of tumor volume in a xenograft model compared with single agent (78). In vitro experiments, two agents caused massive death of HT29 cells under hypoxic condition. However, HCT116 cells did not respond to the treatment probably due to the activating mutations of both the PI3K/Akt and Ras/MAPK pathways in the cells.
Torin 1
Torin 1 was discovered by Gray and Sabatini from a biochemical screening for inhibitors of mTOR in a library of heterocyclic compounds (79). It has much broader substrates than rapamycin. Torin 1 can also inhibit mTORC2 as well as rapamycin-resistant phosphorylation of 4EBP1. Therefore, it is more effective than rapamycin in anti-cancer treatment.
AZD8055 (AstraZeneca)
AZD8055 inhibits both mTORC1 and mTORC2 and has been tested both in vitro and in vivo (80). The IC50 was identified to be 0.8nM. In vitro it inhibited cancer cell proliferation in lung cancer cell lines H838 and A549 with decreased phosphorylation of both mTORC1 and mTORC2 downstream targets such as p70S6K, 4EPB1 and Akt. In animal xenograft models, it led to regression of tumours including colon cancer (80). AZD8055 has also synergistic effect with chemotherapeutic agents and is now in phase I clinical trial (81-83).
OSI-027 (OSI Pharmaceuticals Inc.)
OSI-027 inhibits both mTORC1 and mTORC2 with IC50 22 nM and 65nM respectively (84,85). Preclinical studies showed it inhibited cancer cell proliferation. It also reduced vascular endothelial growth factor (VEGF) production and vessel sprouting (86). OSI-027 is now in aphase I clinical trial for solid tumors and lymphomas (NCT00698243).
INK-128 (Intellikine)
INK-128, the first compound of Intellikine used for clinical trial, is an orally administered mTOR inhibitor which selectively inhibits mTORC1 and mTORC2. It directly inhibits the mTOR kinase in both complexes with the IC50 1nM. The compound is much more potent than rapamycin. In both in vitro and in vivo experiments INK- 128 was shown to be effective for several cancers indicated by reduced phosphorylation of mTORC1 down-stream targets p70S6K1 and 4EPB1, and mTORC2 down-stream target Akt (87). A phase I clinical trial is employed to test the safety and pharmacokinetics of INK-128 in advanced solid tumors (NCT01058707). Another clinical trial is to test its combinational use with paclitaxel and trastuzumab in HER2 positive breast cancers (NCT01351350).
Dual inhibitors of PI3K and mTOR
XL765
XL765 has been tested in several cancers such as pancreatic cancer and glioblastoma cell lines (88,90). It was shown to increase cytotoxicity in a dose-dependent manner in glioblastoma cell lines with the inhibition of the PI3K/Akt pathway (90). The agent had additive effect with chemotherapeutic agent temozolomide. XL765 was also effective in xenograft models. It was shown to induce higher autophagy. The combination of XL765 with autophagy inhibitor chloroquine produced an synergistic effect (88).
SF1126
SF1126 was developed by Garlich et al. from Ly294002 with increased solubility and half-life (91). It has been studied in breast cancer and neuroblastoma (92,93). In HER2 over-expressed breast cancer cells, which are resistant to trastuzumab, SF1126 caused apoptosis, inhibited cell proliferation and colony formation (92). The phosphorylation of Akt was reduced with the increased apoptosis markers caspase 3 activity and PARP protein. In neuroblastoma the compound was shown to cause apoptosis and increase sensitivity to doxorubicin (93). It reduced phophorylation of Akt induced by IGF-1 and disrupted actin cytoskeleton. SF1126 also inhibited tumo growth in an in vivo neuroblastoma xenograft model (93).
PI-103 (Astellas Pharma Inc.)
PI-103 acts on PI3K as well as mTORC1 and mTORC2. It has been shown to inhibit cell proliferation of glioma in vitro and reduce the size of xenograft gliomas in mice with inhibition of mTOR and PI3K kinases (94-97). It was also effective to several other cancers including colon, prostate, hepatoma, acute myeloid leukemia, neuroblastoma and nonsmall cell lung cancers (98-104). PI-103 was shown to have synergistic effects with sorafenib, radiotherapy, arsenic disulfide, rapamycin (100,101,105,106). The compound is rapidly metabolized in vivo, therefore it is not suitable for clinical use but could be used as a lead compound for further development.
BEZ235 (Novartis)
Bez235 is derived from imidazoquinoline and has been studied in many cancer cell lines including colon cancer (49,107-114). BEZ-235 causes transient PI3K blockage and sustained decreases in mTORC1/ mTORC2. In vitro experiments, Bez235 decreased colon cancer cell viability independent of PIK3CA mutations (113). In vivo, treatment with Bez235 decreased xenograft colon cancer volume by 43% compared with a 97% growth in the control (113). There were a 56% decrease in cell proliferation and 75% reduction in angiogenesis but no effect on apoptosis. At present there are 7 clinical trials are recruiting for Bez235.
BGT226 (Novartis)
Anti-cancer effect of BGT226 has been tested in breast cancer and in head and neck cancer (51,115). In estrogen positive breast cancer cell lines, BGT226 had synergistic effects with fulvestrant (51). It also inhibited the proliferation of many head and neck cancer cell lines. The signaling pathway analysis showed reduced phosphorylation of Akt at Ser473 and mTOR at Ser2448 by BGT226. The cells were shown to be arrested in G0/G1 phase with decreased number at S phase. In FaDu cancer cell xenograft mouse model, oral administration of BGT226 at dosages of 2.5 and 5 mg/kg for three weeks inhibited the tumour growth by 34.7% and 76.1%, respectively.
GDC-0980 (Genentech Inc.)
GDC-0980 is developed from GDC-0941 with the additional ability to inhibit mTOR by substituting indazole with aminopyrimidine (116). It is effective against many cancer cell lines but less effective against the cells with activated MAPK pathway (116,117). Oral administration of a low dose of GDC-0980 (1 mg/kg) inhibited tumo growth in a xenograft model (116). It is now in phase I clinical trial.
MAPK pathway inhibitors
MAPK is a mitogenic pathway and plays a key role in cell proliferation and survival and responss to diverse mitogens such as EGFR, erbB2, PDGFR and environmental stresses (118-121). MAPK is activated in many cancers and promotes cancer cell proliferation and decreases apoptosis. Inhibition of the pathway has been shown to be effective to inhibit cancer cell growth. As this pathway has been demonstrated to be activated in obesity-associated colon cancer, its inhibition may be effective for the treatment of the disease (26,43). The details of the pathway has been reviewed else where (120,122). Some inhibitors of the MAPK pathway are listed in Table 2 and described below.
Sorafenib (Bay 43-9006, Nexavar)
Sorafenib, the first RAF inhibitor for clinical trial, was initially developed for the inhibition of RAF and was later found to also inhibit PDGFR, VEGFR1/2, c-kit, FGFR1 and FLT3 (123). The synergistic effect of sorafenib and vorinostat treatment was demonstrated in colon cancer cells SW480, DLD-1 and HCT116 (124). It was shown that pErk1/2 was inhibited but pAkt was not. The authors also demonstrated that pErk was more important in HCT116 cells than pAkt for drug resistance. However, the effect of sorafenib on cells with mutated BRAF is limited. In obesity-associated colon cancer, activation of the MAPK pathway is not caused by gene mutations. Therefore, sorafenib may be effective for the disease.
PLX-4032 (Plexxikon)
PLX-4032 is small molecule inhibiting Raf. It was developed by Plexxikon due to the discovery that 80% of melanomas and 20% of colorectal cancers have BRaf activating mutations. PLX-4032 has been tested in melanoma to be effective (125-127). It increased the sensitivity of melanoma cells to radiotherapy (125). The phase I clinical trial was successful and now phase II and phase III clinical trials are underway (128).
GSK2118436
GSK2118436 was also studied in melanoma (83,129-132). It inhibited mutated B-Raf and increased clinical responses to chemotherapy markedly (83). However the use of GSK2118436 subsequently increased platelet derived growth factor receptor and resulted in the resistance to the treatment. The use of PI3K or mTORC1/2 inhibitors produced synergistic inhibition. Combinational use of MEK1/2, PI3K and mTORC1/2 in melanoma cancer cells triggered profound apoptosis.
RAF265 (Novartis)
RAF265 has been demonstrated to be effective in melanoma and endocrine cancers (133-136). The studies also found that Akt pathway may compensate to cause the resistance to RAF265. Thus dual inhibition approaches were tested (137-139). A phase I for advanced solid tumo with Ras or BRAFV600E mutations is recruited by Novartis (NCT01352273).
MEK1/2 inhibitors
CI-1040 (PD-184352) (Pfizer-oncology)
CI-1040 is the first MEK/12 inhibitor used for clinical trial. But the efficacy is not satisfactory. The compound was tested for several cancers including colon cancer (140-142). The IC50 for colon 26 is 17 nM (142). Pfizer has completed two phase II studies in colon cancer but the outcome is not satisfactory (NCT00034827 and NCT00033384). Therefore, second generation of MEK1/2 inhibitors were developed.
PD-0325901(Pfizer-oncology)
PD-0325901 has been studied in colon cancer in a pilot study (141,143-146). Initially, it was given orally at a dose of 20 mg twice daily for 21 days but changed to 15 mg because it was not well tolerated. The trial was terminated earlier due to side-effects. There is severe neurological toxicity for this compound and thus it is no longer being tested in clinical trials.
AZD6244 (AstraZeneca)
AZD has been tested in colon cancer (). The agent has been used for phase I clinical trials with satisfactory results and the dosage used was well tolerated (150). FOXO3a was found to increase compensatorily (149). A phase II clinical trial for colorectal cancer is supported by AstraZeneca and expected to be completed in December 2011 (www.clinical trials.gov. NCT01116271). In the trial, AZD6244 is used in combination with irinotecan. Another phase I supported by NIH is also in recruiting, AZD6244 is used with cetuximab (NCT01287130).
GSK1120212
GSK1120212 (also called JTP-74057) was discovered by a highthroughput screening for compounds that induced the expression of p15INK4b, which is down-stream of ERK1/2 and inhibited by ERK1/2 (151). GSK1120212 was tested in colon cancer (151-153) and was shown to be much more potent than the second generation MEK1/2 inhibitors AZD6244 and PD0325901. It effectively inhibited MEK1/2 in 9 colon cancer cell lines and decreased cell proliferation with IC50 from 0.92 nm to 3.4 nM. In animal models, the compound reduced HT-29 xenograft tumo sizes (151). GSK1120212 was administered orally daily for 14 days at dosage of 0.3 mg/kg and 1 mg/kg. Both dosages were effective but the higher dose (1 mg/kg) inhibited tumo growth completely. The compound had additive effects with oxaliplatin and 5-FU to cause colon cancer cell apoptosis. The combination with irinotecan metabolite SN-38 also produced synergistic effects. A phase I in combination with Akt inhibitor is recruited by GlaxoSmithKline (NCT01138085).
Signaling molecules | RAF | Mutated RAF | MEK |
Inhibitors | sorafenib | PLX-4032, | AZD6244, |
GSK2118436, | GSK1120212, | ||
RAF265 | CI-1040, | ||
PD-0325901 |
Dual inhibitors of Akt and MAPK
Perfosine (Keryx)
Perfosine (KRX-0401) is an oral anti-cancer agent which inhibits both Akt and MAPK. It acts on the PH domain of Akt and thus prevents Akt to be translocated to plasma membrane for phosphorylation. In a phase II clinical trial, it was very effective against colon cancer with doubled time for metastatic cancer to progress (154). Clinical trials showed its side-effects are fatigues and gastrointestinal toxicity. Perfosine is now in a phase III clinical trial for colorectal cancer in combination with capecitabine by Kerynx (NCT01097018). Perfosine has been approved by FDA to be used in colon cancer treatment.
Erucylphosphocholine
Erucylphosphocholine is derived from perfosine. It has been tested for glioblastoma. It caused decreased proliferation via inhibition of Erk and pro-apoptosis by activation of JNK (155). Erucylphosphocholine has also strong activity against endometrial and ovarian cancer cells (156).
STAT3 Pathway Inhibitors
STAT3 was demonstrated to be important for the carcinogenesis and metastasis of colon cancer (157,158). Inhibition of STAT3 is effective for cancer lines with increased STAT3 pathway but less effective in those cells with low STAT3 activity. In obesity-associated colon cancer, increased leptin has been shown to accelerate colon cancer partially via STAT3 (34). Therefore, inhibition of STAT3 may be used for the targeted therapy for obesity-associated colon cancer. STAT3 inhibitors include NSC74859, LLL12 and stattic.
NSC74859
NSC74859 is a salicylic acid-based inhibitor with a low molecular weight. It was discovered by Turkson through screening of a National Cancer Institute chemical compound library via in silico methods (159). IC50 for the inhibition of the SH2 domain of STAT3 is 86 M. NSC74859 has been shown to reduce the expression of down-stream targets of STAT3 such as cyclin D1, Bcl-xL and surviving (160).
LLL12
LLL 12 was developed by Dr. Pui-Kai Li at Ohio State University (161). It has been shown to induce apoptosis and inhibit cancer cell growth in several cancers including colon colon under stimulation of IL-6 (157,161-168).
Stattic
Stattic is the abbreviation for stat three inhibitory compounds. It was discovered after screening thousands of compounds. Stattic can inhibit the SH2 domain of STAT3 specifically (169). The effectiveness of Stattic has been tested in colon cancer. In ALDH+/CD133+ colon cancer stem cells, STAT3 activity was increased and stattic inhibited STAT3 and its down-stream targets (157). In obesity-associated colon cancer STAT3 pathway may be activated by leptin and IL-6. Therefore, inhibition of STAT3 by Stattic may be an approach for the treatment of the disease.
Selection of approaches to target activated multiple signaling pathways in obesity-associated colon cancer
As discussed above many inhibitors can be chosen to inhibit the activated signaling pathways in obesity-associated colon cancer. Studies have used some of these inhibitors to inhibit signaling molecules such as PI3K or mTOR and showed the effectiveness of tested inhibitors (30-32). Due to multiple factors and multiple signaling pathways are activated in obesity-associated colon cancer, inhibition of a single molecule may be not sufficient for treatment. More options exist to increase treatment efficacy for obesity-associated colon cancer.
Dual inhibitors of PI3K and mTOR such as Bez235 are particularly interesting. mTOR could be highly activated in obesity because it is activated by both the PI3K/Akt and MAPK pathways (26). Therefore, it can be used as a target for inhibition. However, mTOR is known to feedback inhibit the PI3K/Akt pathway. Inhibition of mTOR leads to increases the activity of PI3K/Akt. Dual inhibitors of PI3K/mTOR could abrogate this effect.
Dual inhibitors of Akt and MAPK could also be chosen for the treatment of obesity-associated colon cancer. These drugs inhibit key signaling molecules in two important signaling pathways in obesity and therefore could be more effective in reversing the activated multiple signaling pathways in obesity-associated colon cancer. As several studies have shown that one of the pathways may compensatorily increases when another is inhibited, the use of dual inhibitors may be particularly effective.
Another approach could be the combinational use of two or more inhibitors such as a dual inhibitor of PI3K/mTOR and a MAPK inhibitor. This could maximiz the inhibition of multiple signaling pathways in obesity-associated colon cancer. However, it is not known if the side-effects will also increase. These combinations warrant further investigation in cancer cell lines and animal models. If the results obtained are promising, combinational targeted therapy of obesityassociated colon cancer may be further validated in clinical trials.
Conclusions
Activation of multiple signaling pathways in obesity has been associated with the poorer treatment outcome of obesity-associated colon cancer. Therefore, targeted inhibition of these pathways could increase the efficacy of chemotherapy in this disease. At present, many inhibitors developed by different companies are available to inhibit these pathways. Most studies have tried to inhibit one signaling molecule for the disease with some effectiveness. However, it may be not sufficient to inhibit one signaling molecule for the activation of multiple signaling pathways in obesity-associated colon cancer. There are more options to inhibit several key signaling molecules to increase treatment efficacy for example, Bez235 can inhibit both PI3K and mTOR, perfosine can inhibit both Akt and MAPK. Two inhibitors may be used in combination to further increase treatment efficacy such as a dual inhibitor of PI3K/mTor and a MAPK inhibitor.
References
- Swinburn BA, Sacks G, Hall KD, et al. The global obesity pandemic: shaped by global drivers and local environments. Lancet 2011;378:804-14.[LinkOut]
- Caballero B. The global epidemic of obesity: an overview. Epidemiol Rev 2007;29:1-5.[LinkOut]
- Wu Y. Overweight and obesity in China. BMJ 2006;333:362-3.[LinkOut]
- Shen J, Goyal A, Sperling L. The emerging epidemic of obesity, diabetes, and the metabolic syndrome in china. Cardiol Res Pract 2012;2012:178675.
- Zhang L, Zhang WH, Zhang L, et al. Prevalence of overweight/obesity and its associations with hypertension, diabetes, dyslipidemia, and metabolic syndrome: a survey in the suburban area of Beijing, 2007. Obes Facts 2011;4:284-9.[LinkOut]
- Chen J. The Src/PI3K/Akt signal pathway may play a key role in decreased drug efficacy in obesity-associated cancer. J Cell Biochem 2010;110:279- 80.[LinkOut]
- Huang XF, Chen JZ. Obesity, the PI3K/Akt signal pathway and colon cancer. Obes Rev 2009;10:610-6.[LinkOut]
- Renehan A, Dive C. Obesity insulin and chemoresistance in colon cancer. J Gastrointest Oncol 2011;2:8-10.[LinkOut]
- Renehan AG, Tyson M, Egger M, et al. Body-mass index and incidence of cancer: a systematic review and meta-analysis of prospective observational studies. Lancet 2008;371:569-78.[LinkOut]
- Calle EE, Kaaks R. Overweight, obesity and cancer: epidemiological evidence and proposed mechanisms. Nat Rev Cancer 2004;4:579-91.[LinkOut]
- Gislette T, Chen J. The possible role of IL-17 in obesity-associated cancer. ScientificWorldJournal 2010;10:2265-71.[LinkOut]
- Paz-Filho G, Lim EL, Wong ML, et al. Associations between adipokines and obesity-related cancer. Front Biosci 2011;16:1634-50.[LinkOut]
- Paz-Filho G, Wong ML, Licinio J.Ten years of leptin replacement therapy. Obes Rev 2011;12:e315-23.[LinkOut]
- Chen J, McMillan NA. Molecular basis of pathogenesis, prognosis and therapy in chronic lymphocytic leukaemia. Cancer Biol Ther 2008;7:174-9.[LinkOut]
- Chen J, McMillan NA. Multiple signal pathways in the leukemogenesis and therapeutic implications. Leuk Res 2007;31:1759-60.[LinkOut]
- Chen J, Huang XF. The signal pathways in azoxymethane-induced colon cancer and preventive implications. Cancer Biol Ther 2009;8:1313-7.[LinkOut]
- Hanahan D, Weinberg RA. Hallmarks of cancer: the next generation. Cell 2011;144:646-74.[LinkOut]
- Chen J. Is Src the key to understanding metastasis and developing new treatments for colon cancer? Nat Clin Pract Gastroenterol Hepatol 2008;5:306-7.[LinkOut]
- Vogelstein B, Kinzler KW. Cancer genes and the pathways they control. Nat Med 2004;10:789-99.[LinkOut]
- Jemal A, Bray F, Center MM, et al. Global cancer statistics. CA Cancer J Clin 2011;61:69-90.[LinkOut]
- Fearon ER. Molecular genetics of colorectal cancer. Annu Rev Pathol 2011;6:479-507.[LinkOut]
- Pretlow TP, Pretlow TG. Mutant KRAS in aberrant crypt foci (ACF): initiation of colorectal cancer? Biochim Biophys Acta 2005;1756:83-96.[LinkOut]
- Leary RJ, Lin JC, Cummins J, et al. Integrated analysis of homozygous deletions, focal amplifications, and sequence alterations in breast and colorectal cancers. Proc Natl Acad Sci U S A 2008;105:16224-9.[LinkOut]
- Kinzler KW, Vogelstein B. Lessons from hereditary colorectal cancer. Cell 1996;87:159-70.[LinkOut]
- Chen J, Raymond K. Nuclear receptors, bile-acid detoxification, and cholestasis. Lancet 2006;367:454-6.[LinkOut]
- Chen J. Multiple signal pathways in obesity-associated skin cancer. Toxicol Appl Pharmacol 2010;247:166; author reply 167.[LinkOut]
- Calle EE, Rodriguez C, Walker-Thurmond K, et al. Overweight, obesity, and mortality from cancer in a prospectively studied cohort of U.S. adults. N Engl J Med 2003;348:1625-38.[LinkOut]
- Parr CL, Batty GD, Lam TH, et al. Body-mass index and cancer mortality in the Asia-Pacific Cohort Studies Collaboration: pooled analyses of 424,519 participants. Lancet Oncol 2010;11:741-52.[LinkOut]
- Renehan AG. Obesity and cancer in Asia-Pacific populations. Lancet Oncol 2010;11:704-5.[LinkOut]
- Chen J, Katsifis A, Hu C, et al. Insulin decreases therapeutic efficacy in colon cancer cell line HT29 via the activation of the PI3K/Akt pathway. Curr Drug Discov Technol 2011;8:119-25.[LinkOut]
- Chen J, Huang XF, Qiao L, et al. Insulin caused drug resistance to oxaliplatin in colon cancer cell HT29. J Gastrointest Oncol 2011;2:27-33.[LinkOut]
- Bartucci M, Svensson S, Ricci-Vitiani L, et al. Obesity hormone leptin induces growth and interferes with the cytotoxic effects of 5-fluorouracil in colorectal tumor stem cells. Endocr Relat Cancer 2010;17:823-33.[LinkOut]
- Drew JE. Molecular mechanisms linking adipokines to obesity-related colon cancer: focus on leptin. Proc Nutr Soc 2011:1-6.[LinkOut]
- Endo H, Hosono K, Uchiyama T, et al. Leptin acts as a growth factor for colorectal tumours at stages subsequent to tumour initiation in murine colon carcinogenesis. Gut 2011;60:1363-71.[LinkOut]
- Jaffe T, Schwartz B. Leptin promotes motility and invasiveness in human colon cancer cells by activating multiple signal-transduction pathways. Int J Cancer 2008;123:2543-56.[LinkOut]
- Ratke J, Entschladen F, Niggemann B, et al. Leptin stimulates the migration of colon carcinoma cells by multiple signaling pathways. Endocr Relat Cancer 2010;17:179-89.[LinkOut]
- Sălăgeanu A, Tucureanu C, Lerescu L, et al. Serum levels of adipokines resistin and leptin in patients with colon cancer. J Med Life 2010;3:416-20.[LinkOut]
- Kalaany NY, Sabatini DM. Tumours with PI3K activation are resistant to dietary restriction. Nature 2009;458:725-31.[LinkOut]
- Liu P, Cheng H, Roberts TM, et al. Targeting the phosphoinositide 3-kinase pathway in cancer. Nat Rev Drug Discov 2009;8:627-44.[LinkOut]
- Wee S, Jagani Z, Xiang KX, et al. PI3K pathway activation mediates resistance to MEK inhibitors in KRAS mutant cancers. Cancer Res 2009;69:4286-93.[LinkOut]
- Algire C, Amrein L, Zakikhani M, et al. Metformin blocks the stimulative effect of a high-energy diet on colon carcinoma growth in vivo and is associated with reduced expression of fatty acid synthase. Endocr Relat Cancer 2010;17:351-60.[LinkOut]
- Moore T, Beltran L, Carbajal S, et al. Dietary energy balance modulates signaling through the Akt/mammalian target of rapamycin pathways in multiple epithelial tissues. Cancer Prev Res (Phila) 2008;1:65-76.[LinkOut]
- Park SY, Kim JS, Seo YR, et al. Effects of diet-induced obesity on colitisassociated colon tumor formation in A/J mice. Int J Obes (Lond) 2011. [Epub ahead of print].
- Ihle NT, Williams R, Chow S, et al. Molecular pharmacology and antitumor activity of PX-866, a novel inhibitor of phosphoinositide-3-kinase signaling. Mol Cancer Ther 2004;3:763-72.[LinkOut]
- Williams R, Baker AF, Ihle NT, et al. The skin and hair as surrogate tissues for measuring the target effect of inhibitors of phosphoinositide-3-kinase signaling. Cancer Chemother Pharmacol 2006;58:444-50.[LinkOut]
- Ihle NT, Lemos R, Schwartz D, et al. Peroxisome proliferator-activated receptor gamma agonist pioglitazone prevents the hyperglycemia caused by phosphatidylinositol 3-kinase pathway inhibition by PX-866 without affecting antitumor activity. Mol Cancer Ther 2009;8:94-100.[LinkOut]
- Shapiro G, Kwak E, Baselga J, et al. Beyond mTORC1 - New PI3 Kinase Pathway Inhibitors. J Clin Oncol Suppl 2009;27:s15.
- Chakrabarty A, Snchez V, Kuba MG, et al. Feedback upregulation of HER3 (ErbB3) expression and activity attenuates antitumor effect of PI3K inhibitors. Proc Natl Acad Sci U S A 2011. [Epub ahead of print].
- Aziz SA, Jilaveanu LB, Zito C, et al. Ver tical targeting of the phosphatidylinositol-3 kinase pathway as a strategy for treating melanoma. Clin Cancer Res 2010;16:6029-39.[LinkOut]
- Buonamici S, Williams J, Morrissey M, et al. Interfering with resistance to smoothened antagonists by inhibition of the PI3K pathway in medulloblastoma. Sci Transl Med 2010;2:51ra70.
- Sanchez CG, Ma CX, Crowder RJ, et al. Preclinical modeling of combined phosphatidylinositol-3-kinase inhibition with endocrine therapy for estrogen receptor-positive breast cancer. Breast Cancer Res 2011;13:R21.[LinkOut]
- Kong D, Dan S, Yamazaki K, et al. Inhibition profiles of phosphatidylinositol 3-kinase inhibitors against PI3K superfamily and human cancer cell line panel JFCR39. Eur J Cancer 2010;46:1111-21.[LinkOut]
- Salphati L, Wong H, Belvin M, et al. Pharmacokinetic-pharmacodynamic modeling of tumor growth inhibition and biomarker modulation by the novel phosphatidylinositol 3-kinase inhibitor GDC-0941. Drug Metab Dispos 2010;38:1436-42.[LinkOut]
- Zheng L, Yang W, Zhang C, et al. GDC-0941 sensitizes breast cancer to ABT-737 in vitro and in vivo through promoting the degradation of Mcl-1. Cancer Lett 2011;309:27-36.[LinkOut]
- Herman SE, Gordon AL, Wagner AJ, et al. Phosphatidylinositol 3-kinase- inhibitor CAL-101 shows promising preclinical activity in chronic lymphocytic leukemia by antagonizing intrinsic and extrinsic cellular survival signals. Blood 2010;116:2078-88.[LinkOut]
- Herman SE, Lapalombel la R , Gordon AL, et al . The role of phosphatidylinositol 3-kinase- in the immunomodulatory effects of lenalidomide in chronic lymphocytic leukemia. Blood 2011;117:4323-7.[LinkOut]
- Hoellenriegel J, Meadows SA, Sivina M, et al. The phosphoinositide 3'-kinase delta inhibitor, CAL-101, inhibits B-cell receptor signaling and chemokine networks in chronic lymphocytic leukemia. Blood 2011;118:3603-12.[LinkOut]
- Ikeda H, Hideshima T, Fulciniti M, et al. PI3K/p110{delta} is a novel therapeutic target in multiple myeloma. Blood 2010;116:1460-8.[LinkOut]
- Kahl BS, Cheson BD, Friedberg JW. Clinical Roundtable Monograph: current treatment options for NHL patients refractory to standard therapy: recent data in single-agent and combination therapy. Clin Adv Hematol Oncol 2010;8:1-16.[LinkOut]
- Lannutti BJ, Meadows SA, Herman SE, et al. CAL-101, a p110delta selective phosphatidylinositol-3-kinase inhibitor for the treatment of B-cell malignancies, inhibits PI3K signaling and cellular viability. Blood 2011;117:591-4.[LinkOut]
- Lin TS. New agents in chronic lymphocytic leukemia. Curr Hematol Malig Rep 2010;5:29-34.[LinkOut]
- Parekh S, Weniger MA, Wiestner A. New molecular targets in mantle cell lymphoma. Semin Cancer Biol 2011. [Epub ahead of print].
- Song G, Ouyang G, Bao S. The activation of Akt/PKB signaling pathway and cell survival. J Cell Mol Med 2005;9:59-71.[LinkOut]
- Sharma AK, Sharma A, Desai D, et al. Synthesis and anticancer activity comparison of phenylalkyl isoselenocyanates with corresponding naturally occurring and synthetic isothiocyanates. J Med Chem 2008;51:7820-6.[LinkOut]
- Sharma AK, Kline CL, Berg A, et al. The Akt inhibitor ISC-4 activates prostate apoptosis response protein-4 and reduces colon tumor growth in a nude mouse model. Clin Cancer Res 2011;17:4474-83.[LinkOut]
- Yap TA, Yan L, Patnaik A, et al. First-in-Man Clinical Trial of the Oral Pan- AKT Inhibitor MK-2206 in Patients With Advanced Solid Tumors. J Clin Oncol 2011. [Epub ahead of print].
- Hirai H, Sootome H, Nakatsuru Y, et al. MK-2206, an allosteric Akt inhibitor, enhances antitumor efficacy by standard chemotherapeutic agents or molecular targeted drugs in vitro and in vivo. Mol Cancer Ther 2010;9:1956-67.[LinkOut]
- Brown EJ, Albers MW, Shin TB, et al. A mammalian protein targeted by G1-arresting rapamycin-receptor complex. Nature 1994;369:756-8.[LinkOut]
- Chiu MI, Katz H, Berlin V. RAPT1, a mammalian homolog of yeast Tor, interacts with the FKBP12/rapamycin complex. Proc Natl Acad Sci U S A 1994;91:12574-8.[LinkOut]
- Sabatini DM, Erdjument-Bromage H, Lui M, et al. RAFT1: a mammalian protein that binds to FKBP12 in a rapamycin-dependent fashion and is homologous to yeast TORs. Cell 1994;78:35-43.[LinkOut]
- Hay N, Sonenberg N. Upstream and downstream of mTOR. Genes Dev 2004;18:1926-45.[LinkOut]
- Sparks CA, Guertin DA. Targeting mTOR: prospects for mTOR complex 2 inhibitors in cancer therapy. Oncogene 2010;29:3733-44.[LinkOut]
- Koehl GE, Spitzner M, Ousingsawat J, et al. Rapamycin inhibits oncogenic intestinal ion channels and neoplasia in APC(Min/+) mice. Oncogene 2010;29:1553-60.[LinkOut]
- Gulhati P, Bowen KA, Liu J, et al. mTORC1 and mTORC2 regulate EMT, motility, and metastasis of colorectal cancer via RhoA and Rac1 signaling pathways. Cancer Res 2011;71:3246-56.[LinkOut]
- Schenone S, Brullo C, Musumeci F, et al. ATP-competitive inhibitors of mTOR: an update. Curr Med Chem 2011;18:2995-3014.[LinkOut]
- Benoit M, Dormond-Meuwly A, Demartines N, et al. Targeting the JNK signaling pathway potentiates the antiproliferative efficacy of rapamycin in LS174T colon cancer cells. J Surg Res 2011;167:e193-8.[LinkOut]
- Buck E, Eyzaguirre A, Brown E, et al. Rapamycin synergizes with the epidermal growth factor receptor inhibitor erlotinib in non-small-cell lung, pancreatic, colon, and breast tumors. Mol Cancer Ther 2006;5:2676-84.[LinkOut]
- Pencreach E, Gurin E, Nicolet C, et al. Marked activity of irinotecan and rapamycin combination toward colon cancer cells in vivo and in vitro is mediated through cooperative modulation of the mammalian target of rapamycin/hypoxia-inducible factor-1alpha axis. Clin Cancer Res 2009;15:1297-307.[LinkOut]
- Thoreen CC, Kang SA, Chang JW, et al. An ATP-competitive mammalian target of rapamycin inhibitor reveals rapamycin-resistant functions of mTORC1. J Biol Chem 2009;284:8023-32.[LinkOut]
- Chresta CM, Davies BR, Hickson I, et al. AZD8055 is a potent, selective, and orally bioavailable ATP-competitive mammalian target of rapamycin kinase inhibitor with in vitro and in vivo antitumor activity. Cancer Res 2010;70:288-98.[LinkOut]
- Jiang Q, Weiss JM, Back T, et al. mTOR kinase inhibitor AZD8055 enhances the immunotherapeutic activity of an agonist CD40 antibody in cancer treatment. Cancer Res 2011;71:4074-84.[LinkOut]
- Shao H, Gao C, Tang H, et al. Dual targeting of mTORC1/C2 complexes enhances histone deacetylase inhibitor-mediated anti-tumor efficacy in primary HCC cancer in vitro and in vivo. J Hepatol 2011. [Epub ahead of print].
- Shi H, Kong X, Ribas A, et al. Combinatorial treatments that overcome PDGFR-driven resistance of melanoma cells to V600EB-RAF inhibition. Cancer Res 2011;71:5067-74.[LinkOut]
- Bhagwat SV, Gokhale PC, Crew AP, et al. Preclinical characterization of OSI-027, a potent and selective inhibitor of mTORC1 and mTORC2: distinct from rapamycin. Mol Cancer Ther 2011;10:1394-406.[LinkOut]
- Carayol N, Vakana E, Sassano A, et al. Critical roles for mTORC2- and rapamycin-insensitive mTORC1-complexes in growth and survival of BCRABL- expressing leukemic cells. Proc Natl Acad Sci U S A 2010;107:12469- 74.[LinkOut]
- Falcon BL, Barr S, Gokhale PC, et al. Reduced VEGF production, angiogenesis, and vascular regrowth contribute to the antitumor properties of dual mTORC1/mTORC2 inhibitors. Cancer Res 2011;71:1573-83.[LinkOut]
- Jessen K, Wang SY, Kessler L, et al. Abstract B148: INK128 is a potent and selective TORC1/2 inhibitor with broad oral antitumor activity. Molecular Cancer Therapeutics 2009;8:sB148.
- Mirzoeva OK, Hann B, Hom YK, et al. Autophagy suppression promotes apoptotic cell death in response to inhibition of the PI3K-mTOR pathway in pancreatic adenocarcinoma. J Mol Med (Berl) 2011;89:877-89.
- Molckovsky A, Siu LL. First-in-class, first-in-human phase I results of targeted agents: highlights of the 2008 American society of clinical oncology meeting. J Hematol Oncol 2008;1:20.[LinkOut]
- Prasad G, Sottero T, Yang X, et al. Inhibition of PI3K/mTOR pathways in glioblastoma and implications for combination therapy with temozolomide. Neuro Oncol 2011;13:384-92.[LinkOut]
- Garlich JR, De P, Dey N, et al. A vascular targeted pan phosphoinositide 3-kinase inhibitor prodrug, SF1126, with antitumor and antiangiogenic activity. Cancer Res 2008;68:206-15.[LinkOut]
- Ozbay T, Durden DL, Liu T, et al. In vitro evaluation of pan-PI3-kinase inhibitor SF1126 in trastuzumab-sensitive and trastuzumab-resistant HER2-over-expressing breast cancer cells. Cancer Chemother Pharmacol 2010;65:697-706.[LinkOut]
- Peirce SK, Findley HW, Prince C, et al. The PI-3 kinase-Akt-MDM2- survivin signaling axis in high-risk neuroblastoma: a target for PI-3 kinase inhibitor intervention. Cancer Chemother Pharmacol 2011 Aug 68:325- 35.[LinkOut]
- Fan QW, Knight ZA, Goldenberg DD, et al. A dual PI3 kinase/mTOR inhibitor reveals emergent efficacy in glioma. Cancer Cell 2006;9:341-9.[LinkOut]
- Bagci-Onder T, Wakimoto H, Anderegg M, et al. A dual PI3K/mTOR inhibitor, PI-103, cooperates with stem cell-delivered TRAIL in experimental glioma models. Cancer Res 2011;71:154-63.[LinkOut]
- Westhoff MA, Kandenwein JA, Karl S, et al. The pyridinylfuranopyrimidine inhibitor, PI-103, chemosensitizes glioblastoma cells for apoptosis by inhibiting DNA repair. Oncogene 2009;28:3586-96.[LinkOut]
- Raynaud FI, Eccles SA, Patel S, et al. Biological properties of potent inhibitors of class I phosphatidylinositide 3-kinases: from PI-103 through PI-540, PI-620 to the oral agent GDC-0941. Mol Cancer Ther 2009;8:1725-38.[LinkOut]
- Opel D, Naumann I, Schneider M, et al. Targeting aberrant PI3K/Akt activation by PI103 restores sensitivity to TRAIL-induced apoptosis in neuroblastoma. Clin Cancer Res 2011;17:3233-47.[LinkOut]
- Al-Saffar NM, Jackson LE, Raynaud FI, et al. The phosphoinositide 3-kinase inhibitor PI-103 downregulates choline kinase alpha leading to phosphocholine and total choline decrease detected by magnetic resonance spectroscopy. Cancer Res 2010;70:5507-17.[LinkOut]
- Gedaly R, Angulo P, Hundley J, et al. PI-103 and sorafenib inhibit hepatocellular carcinoma cell proliferation by blocking Ras/Raf/MAPK and PI3K/AKT/mTOR pathways. Anticancer Res 2010;30:4951-8.[LinkOut]
- Hong Z, Xiao M, Yang Y, et al. Arsenic disulfide synergizes with the phosphoinositide 3-kinase inhibitor PI-103 to eradicate acute myeloid leukemia stem cells by inducing differentiation. Carcinogenesis 2011;32:1550-8.[LinkOut]
- Kojima K, Shimanuki M, Shikami M, et al. The dual PI3 kinase/mTOR inhibitor PI-103 prevents p53 induction by Mdm2 inhibition but enhances p53-mediated mitochondrial apoptosis in p53 wild-type AML. Leukemia 2008;22:1728-36.[LinkOut]
- Park S, Chapuis N, Bardet V, et al. PI-103, a dual inhibitor of Class IA phosphatidylinositide 3-kinase and mTOR, has antileukemic activity in AML. Leukemia 2008;22:1698-706.[LinkOut]
- Zou ZQ, Zhang XH, Wang F, et al. A novel dual PI3Kalpha/mTOR inhibitor PI-103 with high antitumor activity in non-small cell lung cancer cells. Int J Mol Med 2009;24:97-101.[LinkOut]
- Mazzoletti M, Bortolin F, Brunelli L, et al. Combination of PI3K/mTOR inhibitors: antitumor activity and molecular correlates. Cancer Res 2011;71:4573-84.[LinkOut]
- Prevo R, Deutsch E, Sampson O, et al. Class I PI3 kinase inhibition by the pyridinylfuranopyrimidine inhibitor PI-103 enhances tumor radiosensitivity. Cancer Res 2008;68:5915-23.[LinkOut]
- Awasthi N, Yen PL, Schwarz MA, et al. The efficacy of a novel, dual PI3K/mTOR inhibitor NVP-BEZ235 to enhance chemotherapy and antiangiogenic response in pancreatic cancer. J Cell Biochem 2011.[LinkOut]
- Bhende PM, Park SI, Lim MS, et al. The dual PI3K/mTOR inhibitor, NVP-BEZ235, is efficacious against follicular lymphoma. Leukemia 2010;24:1781-4.[LinkOut]
- Chapuis N, Tamburini J, Green AS, et al. Dual inhibition of PI3K and mTORC1/2 signaling by NVP-BEZ235 as a new therapeutic strategy for acute myeloid leukemia. Clin Cancer Res 2010;16:5424-35.[LinkOut]
- Chiarini F, Grimaldi C, Ricci F, et al. Activity of the novel dual phosphatidylinositol 3-kinase/mammalian target of rapamycin inhibitor NVP-BEZ235 against T-cell acute lymphoblastic leukemia. Cancer Res 2010;70:8097-107.[LinkOut]
- Cho DC, Cohen MB, Panka DJ, et al. The efficacy of the novel dual PI3- kinase/mTOR inhibitor NVP-BEZ235 compared with rapamycin in renal cell carcinoma. Clin Cancer Res 2010;16:3628-38.[LinkOut]
- Elfiky AA, Aziz SA, Conrad PJ, et al. Characterization and targeting of phosphatidylinositol-3 kinase (PI3K) and mammalian target of rapamycin (mTOR) in renal cell cancer. J Transl Med 2011;9:133.[LinkOut]
- Roper J, Richardson MP, Wang WV, et al. The dual PI3K/mTOR inhibitor NVP-BEZ235 induces tumor regression in a genetically engineered mouse model of PIK3CA wild-type colorectal cancer. PLoS One 2011;6:e25132.[LinkOut]
- Santiskulvong C, Konecny GE, Fekete M, et al. Dual targeting of phosphoinositide 3-kinase and mammalian target of rapamycin using NVPBEZ235 as a novel therapeutic approach in human ovarian carcinoma. Clin Cancer Res 2011;17:2373-84.[LinkOut]
- Chang KY, Tsai SY, Wu CM, et al. Novel Phosphoinositide 3-Kinase/ mTOR Dual Inhibitor, NVP-BGT226, Displays Potent Growth-Inhibitory Activity against Human Head and Neck Cancer Cells In Vitro and In Vivo. Clin Cancer Res 2011;17:7116-26[LinkOut]
- Sutherlin DP, Bao L, Berry M, et al. Discovery of a Potent, Selective, and Orally Available Class I Phosphatidylinositol 3-Kinase (PI3K)/Mammalian Target of Rapamycin (mTOR) Kinase Inhibitor (GDC-0980) for the Treatment of Cancer. J Med Chem 2011;54:7579-87.[LinkOut]
- Wallin JJ, Edgar KA, Guan J, et al. GDC-0980 is a novel class I PI3K/mTOR kinase inhibitor with robust activity in cancer models driven by the PI3K pathway. Mol Cancer Ther 2011. [Epub ahead of print].[LinkOut]
- Sebolt-Leopold JS. Advances in the development of cancer therapeutics directed against the RAS-mitogen-activated protein kinase pathway. Clin Cancer Res 2008;14:3651-6.[LinkOut]
- Sebolt-Leopold JS, English JM. Mechanisms of drug inhibition of signalling molecules. Nature 2006;441:457-62.[LinkOut]
- Sebolt-Leopold JS, Herrera R. Targeting the mitogen-activated protein kinase cascade to treat cancer. Nat Rev Cancer 2004;4:937-47.[LinkOut]
- Blume-Jensen P, Hunter T. Oncogenic kinase signalling. Nature 2001;411:355-65.[LinkOut]
- Wagner EF, Nebreda AR. Signal integration by JNK and p38 MAPK pathways in cancer development. Nat Rev Cancer 2009;9:537-49.[LinkOut]
- Wilhelm SM, Adnane L, Newell P. Preclinical overview of sorafenib, a multikinase inhibitor that targets both Raf and VEGF and PDGF receptor tyrosine kinase signaling. Mol Cancer Ther 2008;7:3129-40.[LinkOut]
- Walker T, Mitchell C, Park MA, et al. Sorafenib and vorinostat kill colon cancer cells by CD95-dependent and -independent mechanisms. Mol Pharmacol 2009;76:342-55.[LinkOut]
- Sambade MJ, Peters EC, Thomas NE, et al. Melanoma cells show a heterogeneous range of sensitivity to ionizing radiation and are radiosensitized by inhibition of B-RAF with PLX-4032. Radiother Oncol 2011;98:394-9.[LinkOut]
- Smalley KS. PLX-4032, a small-molecule B-Raf inhibitor for the potential treatment of malignant melanoma. Curr Opin Investig Drugs 2010;11:699- 706.[LinkOut]
- Flaherty KT, Puzanov I, Kim KB, et al. Inhibition of mutated, activated BRAF in metastatic melanoma. N Engl J Med 2010;363:809-19.[LinkOut]
- Brower V. BRAF inhibitors: research accelerates in wake of positive findings. J Natl Cancer Inst 2010;102:214-5.[LinkOut]
- Dienstmann R, Tabernero J. BRAF as a target for cancer therapy. Anticancer Agents Med Chem 2011;11:285-95.[LinkOut]
- Hong S, Hong S, Han SB. Overcoming metastatic melanoma with BRAF inhibitors. Arch Pharm Res 2011;34:699-701.[LinkOut]
- Pratilas CA, Xing F, Solit DB. Targeting Oncogenic BRAF in Human Cancer. Curr Top Microbiol Immunol 2011. [Epub ahead of print].[LinkOut]
- Ribas A, Flaherty KT. BRAF targeted therapy changes the treatment paradigm in melanoma. Nat Rev Clin Oncol 2011;8:426-33.[LinkOut]
- Chen J, Shen Q, Labow M, et al. Protein kinase D3 sensitizes RAF inhibitor RAF265 in melanoma cells by preventing reactivation of MAPK signaling. Cancer Res 2011;71:4280-91.[LinkOut]
- Hersey P, Bastholt L, Chiarion-Sileni V, et al. Small molecules and targeted therapies in distant metastatic disease. Ann Oncol 2009;20:svi35-40.[LinkOut]
- Tseng JR, Stuart D, Aardalen K, et al. Use of DNA microarray and small animal positron emission tomography in preclinical drug evaluation of RAF265, a novel B-Raf/VEGFR-2 inhibitor. Neoplasia 2011;13:266-75.[LinkOut]
- Zitzmann K, de Toni E, von Rden J, et al. The novel Raf inhibitor Raf265 decreases Bcl-2 levels and confers TRAIL-sensitivity to neuroendocrine tumour cells. Endocr Relat Cancer 2011;18:277-85.[LinkOut]
- Jin N, Jiang T, Rosen DM, et al. Synergistic Action of a RAF Inhibitor and a Dual PI3K/mTOR Inhibitor in Thyroid Cancer. Clin Cancer Res 2011;17:6482-9.[LinkOut]
- Mordant P, Loriot Y, Leteur C, et al. Dependence on phosphoinositide 3-kinase and RAS-RAF pathways drive the activity of RAF265, a novel RAF/VEGFR2 inhibitor, and RAD001 (Everolimus) in combination. Mol Cancer Ther 2010;9:358-68.[LinkOut]
- Zitzmann K, Rden J, Brand S, et al. Compensatory activation of Akt in response to mTOR and Raf inhibitors - a rationale for dual-targeted therapy approaches in neuroendocrine tumor disease. Cancer Lett 2010;295:100-9.[LinkOut]
- Allen LF, Sebolt-Leopold J, Meyer MB. CI-1040 (PD184352), a targeted signal transduction inhibitor of MEK (MAPKK). Semin Oncol 2003;30:105-16.[LinkOut]
- Rinehart J, Adjei AA, Lorusso PM, et al. Multicenter phase II study of the oral MEK inhibitor, CI-1040, in patients with advanced non-small-cell lung, breast, colon, and pancreatic cancer. J Clin Oncol 2004;22:4456-62.[LinkOut]
- Sebolt-Leopold JS, Dudley DT, Herrera R, et al. Blockade of the MAP kinase pathway suppresses growth of colon tumors in vivo. Nat Med 1999;5:810-6.[LinkOut]
- Boasberg PD, Redfern CH, Daniels GA, et al. Pilot study of PD-0325901 in previously treated patients with advanced melanoma, breast cancer, and colon cancer. Cancer Chemother Pharmacol 2011;68:547-52.[LinkOut]
- Halilovic E, She QB, Ye Q, et al. PIK3CA mutation uncouples tumor growth and cyclin D1 regulation from MEK/ERK and mutant KRAS signaling. Cancer Res 2010;70:6804-14.[LinkOut]
- Leyton J, Smith G, Lees M, et al. Noninvasive imaging of cell proliferation following mitogenic extracellular kinase inhibition by PD0325901. Mol Cancer Ther 2008;7:3112-21.[LinkOut]
- Wee S, Jagani Z, Xiang KX, et al. PI3K pathway activation mediates resistance to MEK inhibitors in KRAS mutant cancers. Cancer Res 2009;69:4286-93.[LinkOut]
- Dry JR, Pavey S, Pratilas CA, et al. Transcriptional pathway signatures predict MEK addiction and response to selumetinib (AZD6244). Cancer Res 2010;70:2264-73.[LinkOut]
- Shannon AM, Telfer BA, Smith PD, et al. The mitogen-activated protein/ extracellular signal-regulated kinase kinase 1/2 inhibitor AZD6244 (ARRY- 142886) enhances the radiation responsiveness of lung and colorectal tumor xenografts. Clin Cancer Res 2009;15:6619-29.[LinkOut]
- Yang JY, Chang CJ, Xia W, et al. Activation of FOXO3a is sufficient to reverse mitogen-activated protein/extracellular signal-regulated kinase kinase inhibitor chemoresistance in human cancer. Cancer Res 2010;70:4709-18.[LinkOut]
- Adjei AA, Cohen RB, Franklin W, et al. Phase I pharmacokinetic and pharmacodynamic study of the oral, small-molecule mitogen-activated protein kinase kinase 1/2 inhibitor AZD6244 (ARRY-142886) in patients with advanced cancers. J Clin Oncol 2008;26:2139-46.[LinkOut]
- Yamaguchi T, Kakefuda R, Tajima N, et al. Antitumor activities of JTP 74057 (GSK1120212), a novel MEK1/2 inhibitor, on colorectal cancer cell lines in vitro and in vivo. Int J Oncol 2011;39:23-31.[LinkOut]
- Dimou AT, Syrigos KN, Saif MW. Novel agents in the management of pancreatic adenocarcinoma: phase I studies. Highlights from the "2011 ASCO Gastrointestinal Cancers Symposium". San Francisco, CA, USA. January 20-22, 2011. JOP 2011;12:114-6.[LinkOut]
- Gilmartin AG, Bleam MR, Groy A, et al. GSK1120212 (JTP-74057) is an inhibitor of MEK activity and activation with favorable pharmacokinetic properties for sustained in vivo pathway inhibition. Clin Cancer Res 2011;17:989-1000.[LinkOut]
- Keryx. Survival benefit with KRX-0401 in mCRC treatment. Smartoncology 2010;8.
- Kugler W, Erdlenbruch B, Otten K, et al. MAP kinase pathways involved in glioblastoma response to erucylphosphocholine. Int J Oncol 2004;25:1721- 7.[LinkOut]
- Takai N, Ueda T, Nasu K, et al. Erucylphosphocholine shows a strong antigrowth activity in human endometrial and ovarian cancer cells. Gynecol Oncol 2008;111:336-43.[LinkOut]
- Lin L, Liu A, Peng Z, et al. STAT3 is necessary for proliferation and survival in colon cancer-initiating cells. Cancer Res 2011. [Epub ahead of print].[LinkOut]
- Yu H, Pardoll D, Jove R. STATs in cancer inflammation and immunity: a leading role for STAT3. Nat Rev Cancer 2009;9:798-809.[LinkOut]
- Siddiquee K, Zhang S, Guida WC, et al. Selective chemical probe inhibitor of Stat3, identified through structure-based virtual screening, induces antitumor activity. Proc Natl Acad Sci U S A 2007;104:7391-6.[LinkOut]
- Zhang X, Yue P, Fletcher S, et al. A novel small-molecule disrupts Stat3 SH2 domain-phosphotyrosine interactions and Stat3-dependent tumor processes. Biochem Pharmacol 2010;79:1398-409.[LinkOut]
- Lin L, Hutzen B, Li PK, et al. A novel small molecule, LLL12, inhibits STAT3 phosphorylation and activities and exhibits potent growthsuppressive activity in human cancer cells. Neoplasia 2010;12:39-50.[LinkOut]
- Ball S, Li C, Li PK, et al. The small molecule, LLL12, inhibits STAT3 phosphorylation and induces apoptosis in medulloblastoma and glioblastoma cells. PLoS One 2011;6:e18820.[LinkOut]
- Lin L, Benson DM Jr, Deangelis S, et al. A small molecule, LLL12 inhibits constitutive STAT3 and IL-6-induced STAT3 signaling and exhibits potent growth suppressive activity in human multiple myeloma cells. Int J Cancer 2011. [Epub ahead of print].[LinkOut]
- Liu A, Liu Y, Li PK, et al. LLL12 inhibits endogenous and exogenous interleukin-6-induced STAT3 phosphorylation in human pancreatic cancer cells. Anticancer Res 2011;31:2029-35.[LinkOut]
- Liu Y, Fuchs J, Li C, et al. IL-6, a risk factor for hepatocellular carcinoma: FLLL32 inhibits IL-6-induced STAT3 phosphorylation in human hepatocellular cancer cells. Cell Cycle 2010;9:3423-7.[LinkOut]
- Liu Y, Li PK, Li C, et al. Inhibition of STAT3 signaling blocks the antiapoptotic activity of IL-6 in human liver cancer cells. J Biol Chem 2010;285:27429-39.[LinkOut]
- Onimoe GI, Liu A, Lin L, et al. Small molecules, LLL12 and FLLL32, inhibit STAT3 and exhibit potent growth suppressive activity in osteosarcoma cells and tumor growth in mice. Invest New Drugs 2011. [Epub ahead of print].[LinkOut]
- Wei CC, Ball S, Lin L, et al. Two small molecule compounds, LLL12 and FLLL32, exhibit potent inhibitory activity on STAT3 in human rhabdomyosarcoma cells. Int J Oncol 2011;38:279-85.[LinkOut]
- Schust J, Sperl B, Hollis A, et al. Stattic: a small-molecule inhibitor of STAT3 activation and dimerization. Chem Biol 2006;13:1235-42.[LinkOut]