VEGF and cholangiocarcinoma: Feeding the tumor
Abstract
The ability for cells to establish a functional and integrated vascular system is critical for proper tissue growth and cellular homeostasis. Blood vessels provide the essential nutrition and oxygen to cells, however the formation of new blood vessels can also trigger a detrimental and fatal pathway as tumor cells seek out nutritional support. Tumor vasculature and the angiogenic process provide malignant cells with the fuel that they need to spread, invade and metastasize into other organs. "Smart" tumors are able to develop their own blood supply via angiogenic sprouting and vessel formation. Vascular endothelial growth factor (VEGF) has been targeted as a critical regulator of new blood vessel formation (angiogenesis) in numerous cancers including the fatal biliary cancer, cholangiocarcinoma. Cholangiocarcinoma is a cancer of bile ducts or cholangiocytes that is difficult to diagnose and has limited treatment options. Cholangiocytes and cholangiocarcinoma tumors are nourished by the peribiliary plexus; a vascular bed that influences the regulation of cholangiocyte and cholangiocarcinoma response via VEGF expression. Due to its proximity to the vascular bed, cholangiocarcinoma has the ability to metastasize and therefore is regulated by its ability to activate angiogenesis. Besides VEGF, other growth factors contribute to the angiogenic process and can increase tumor proliferation and cholangiocarcinogenesis, however VEGF appears to be the most prominent factor involved in tumor regulation and angiogenesis. VEGF is regulated by VEGF receptor inhibitors or other signaling pathway inhibitors as well as by specific microRNAs that are able to alter VEGF receptor and protein expression. The aim of our review is to define angiogenesis and the formation of new blood vessels and highlight recent studies involving VEGF and cholangiocarcinoma along with new insights into microRNA regulation of VEGF during tumor growth and development. We will briefly touch on recent clinical studies involving VEGF and cholangiocarcinoma. In this concise review we hope to give the reader a better understanding of the importance of angiogenesis and cholangiocarcinoma tumor growth.
Keywords
Cholangiocarcinoma; angiogenesis; vascular endothelial growth factor
Cholangiocarcinoma
Cholangiocarcinoma (CCA) is cancer that occurs due to the transformation of the epithelial cells that line the bile ducts (cholangiocytes) after they become damaged or inflamed beyond repair (1-3). Tumors are classified as intrahepatic or extrahepatic and can occur in any area of the biliary tree (1,2,4). CCA is the second most common hepatic cancer and is increasing in incidence in the United States (5). CCA has a serious prognosis with a high mortality rate because of the difficulty of early diagnosis and lack of successful treatment strategies (2-4). It is difficult to diagnose because of the prominence of unspecific or silent symptoms and CCA is very invasive of many critical organs, so is often not caught until in the advanced stages (2-4). Often times when CCA is diagnosed, the cancer cells have proliferated to a terminal level (4,6). There is a less than 24 months median survival with a 5-year survival rate of less than 5% (7). In fact, 13% of the worldwide cancer deaths are from these types of malignancies (1). Treatment of CCA is limited by its location and prognosis. Currently, the only curative treatment is surgery (total resection), however only if the cancer is diagnosed early on. Unfortunately, this "treatment" only brings survival rates to 22% and 44% over 5 years (8-11). CCA is regulated through hypermethylation of numerous genes (3,12). miR- 141 (13), NFG- (14) and VEGF-C (3) are overexpressed in CCA samples as well as HDC and histamine receptors (15). Inflammation, primary sclerosing cholangitis, liver flukes, biliary malformations, obesity and other liver disease and damage including hepatitis C and cirrhosis have all been identified as risk factors for CCA (1,3,4,16-21).
Similar to other tumors, cholangiocarcinoma has the ability to metastasize and spread into other organs (22). A tumor's ability to invade other tissues is regulated by cellular events and signaling including epithelial to mesenchymal transition and the breakdown of the extracellular matrix (ECM) by specific proteins like matrix metalloproteinases (23). For tumorigenic cells to be successful and survive in other organs and tissues their growth must be supported through the development of new blood vessels allowing for extra nutrition to be in close proximity (24). In contrast to normal blood vessel formation and sprouting, the complexity of tumor spreading often includes a dysregulation of the usually "normal" processes and signaling pathways (25).
Angiogenesis
Angiogenesis influences tumor growth and this could prove to be especially true with CCA. Cancerous cholangiocytes can stimulate capillary network growth in order to increase the metabolic and transportation support for the cell (26). Within the gastrointestinal tract, and especially in the hepatic system, blood flow is prevalent and in close proximity to the bile duct epithelial cells (27). The blood flow in the hepatic system is through the portal veins and the hepatic artery thus providing an abundance of blood supply able to give rise to new potential growth (27). Sprouting angiogenesis, endothelial cell (EC) sprouting and migration, is the primary method of expanding the vascular network (24). We refer the reader to Figure 1 (reprinted with permission from Macmillan Publishers Ltd: [Nature Reviews Cancer, Tumorigenesis and the angiogenic switch, 2003]) for an elegant representation of tumor sprouting and angiogenesis. Tumor cells activate expression of proangiogenic genes like vascular endothelial growth factor (VEGF) (24). When a tumor is deregulated towards hypoxia, disturbed VEGF gradients trigger ECs to sprout (24,28). VEGF binds to VEGF receptors on the targeted ECs and induce a strong expression signal, which contributes to sprouting of the vascular vessel wall (24,29). VEGF to VEGF receptor affinity increases due to interaction with neurophilins (24). These VEGF receptor expressing ECs, or tip cells, are activated (24). The tip cells are able to sense hypoxia from the tumor cells and lengthen the filopodia projections towards them (24). ECs continue to sense the equilibrium status surrounding the cells, and therefore continue to regulate growth until equilibrated (24,28). The vessel wall gathers strength and permeability through the creation of tight junctions and adherens (24,29). Remodeling angiogenesis follows and the vessels are covered and stabilized by mural cells (24). Typically, following remodeling in normal growth, the newly formed vessels receive blood flow and the rise in oxygen levels shut off the angiogenesis process (24). In cancer cells, this series of events is deregulated (24). Tumor cells constantly signal a hypoxia state because missignaling and morphology disrupt normal processes causing over expression of proangiogenic factors (24,30). This is a never-ending cycle (24).
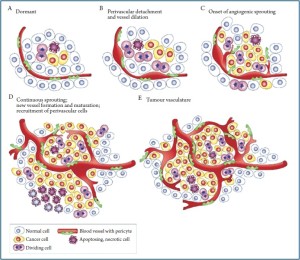
Angiogenic factors
When damage occurs, there are many factors that can regulate the growth of new blood cells or stop current growth. Some factors increase, or activate angiogenesis and there are complementary inhibitors that slow or stop angiogenesis. Both upregulation of activators and downregulation of inhibitors are essential for new growth to occur. Critical mediators and activators of angiogenesis include the growth hormones VEGF, epidermal growth factor (EGF) and nerve growth factor (NGF). Other important angiogenic factors include fibroblast growth factor (FGF), placental growth factor, the angiopoietins and their receptors, Tie1 and Tie2 and finally neuropilin, ephrin and leptin are being recognized as mediators of angiogenesis and tumor growth. Our review will touch briefly on EGF and NGF, but will focus heavily on VEGF as this growth factor has been implicated in numerous studies of cholangiocarcinoma tumorigenesis and angiogenesis.
Growth factors and tumor growth
Research has shown collaborations between angiogenic factors, such as VEGF, and increased aggressive tumor behavior, including prognosis and progression. In patients with cholangiocarcinoma, serum VEGF levels are increased when compared to normal controls (25). Outside of the normal occurrences, pathophysiological states can promote angiogenesis by triggering an increase in angiogenic factors, as is the case with a carcinoma (24,25,29). Tumor cells trigger angiogenesis by increasing the expression of growth factors and growth of tumor cells is directly related to availability of blood (24,30). Cancer cells survive and multiply but are limited in their ability to grow by the amount of nutrients they receive, but with the proper blood supply, tumors can increase growth past the limitations (24,27,29,30). Angiogenesis also plays an important role in metastasis, as metastasis is also affected by blood supply and the availability of supply lends itself to the spread of tumor cells throughout the body (24,30-32).
Angiogenesis and lymphangiogenesis (the process of the formation of lymphatic vessels) are critical regulators in the progression of malignant tumors like cholangiocarcinoma (33). Previous studies have shown that nerve growth factorbeta (NGF-beta) can promote the initiation and progression of many tumors. In addition, VEGF-C has become recognized as an important lymphangiogenic factor (34). A recent immunohistochemical study has shown that nerve growth factor-beta could contribute to the promotion of node metastasis and nerve infiltration in human hilar cholangiocarcinoma (14). Microvessel density (MVD) and lymphatic microvessel density (LVD) are quantitative features of angiogenesis and have been studied extensively as a factor to measure angiogenesis and as a prognostic factor in various malignant tumors. Macrophages and platelet-derived endothelial cell growth factor (PD-ECGF) also play important roles in regulating angiogenesis (35). Secretion of PD-ECGF may contribute to and support cholangiocarcinoma angiogenesis via activation of liver macrophages existing at the invasive margin of cholangiocellular carcinoma (35). In studies of MVD and LVD, a "high" LVD is associated with increased nodal spread, and "high" LVD tumors more frequently develop recurrence (33). These features are found in patients with intrahepatic cholangiocarcinoma (IHC) and may represent a critical function of tumor-associated angiogenesis and lymphangiogenesis during the progression of IHC (33). The anti-inflammatory agent, curcumin has been found to decrease MVD in cholangiocarcinogenesis as well as decreasing tumor proliferation and invasion (36). This study has the potential to be translated into a clinical therapy, as curcumin is a common product that is found naturally.
Estrogens have been shown to work in a coordinated fashion with insulin-like growth factor (IGF1) and its receptor (IGF1-R) to simulate the growth of cholangiocarcinoma (37). Estrogens also stimulate the expression and secretion of VEGF-A, VEGF-C and VEGFRs in cholangiocarcinoma cell lines potentially altering cholangiocarcinoma proliferation and tumor neoangiogenesis (37). Patients with cholangiocarcinoma have an increased amount of IGF1 in their bile when compared to healthy controls (25). It's also been shown that placental growth factor (PGF) expression is increased in intrahepatic cholangiocarcinoma and may assist in the promotion of angiogenesis via liver intestine cadherin (38).
VEGF and cholangiocarcinoma
VEGF is a specific growth hormone that has been shown to have a large role in angiogenesis. VEGF family members include VEGF-A, VEGF-B, VEGF-C and VEGF-D proteins that interact with VEGF receptors. Placental growth factor (PIGF) has also been targeted as a member of the VEGF family that participates in angiogenesis and wound healing, however VEGF-A is the most important and prominent family member involved in angiogenesis. VEGF-C is involved in lymphangiogenesis and VEGF-B is required for embryonic development and angiogenesis. VEGF-A binds to VEGF-R1 and R2, whereas VEGF-C is activated via the VEGF-R2 and R3. Cholangiocytes have been found to secrete VEGF and express the VEGF-R2 and -R3 receptors. During a state of cholangiocyte proliferation (hyperplasia), VEGF secretion and receptor expression is upregulated (39,40). Increased production of VEGF leads to more VEGF present in the surrounding tissue where it binds (via VEGF receptors) to the epithelial cells of the blood vessels. Once bound, VEGF triggers the cells to release numerous factors including matrix metalloproteinases (MMPs) that break down the surrounding extracellular matrix (ECM) (24,28,41). This degeneration creates space for the epithelial cells to divide and migrate into. Once a cell has the ability to migrate, a state of metastasis and invasion begins. This process is detrimental to the organ and surrounding tissue and factors that can disrupt this process could be parlayed into treatment options. Recently, Meng, et al. demonstrated that histamine, via the H4 histamine receptor is able to inhibit the process of MMP upregulation and ECM breakdown thus decreasing metastasis potential in human cholangiocarcinoma (23).
Further studies using histamine and histamine receptors have demonstrated a critical role for this biogenic amine during cholangiocarcinoma progression via interaction with VEGF. Using an H3 histamine receptor agonist, Francis and Alpini, et al. demonstrated that stimulation of this inhibitory receptor induces an activation of protein kinase C alpha with subsequent inhibition of cholangiocarcinoma growth both in vitro and in vivo (42). Important to our discussion in this review, the H3 histamine receptor agonist decreased the expression of both VEGF-A and VEGF-C along with the VEGF receptors, VEGF-R2 and VEGF-R3 thus demonstrating a potential role for inhibition of angiogenesis during cholangiocarcinogenesis (42). Most recently, the enzyme histidine decarboxylase (HDC) that catalyzes histidine formation into histamine, has been found to be overexpressed in human cholangiocarcinoma that correlated with a significant increase in the angiogenic factors, VEGF-A and VEGF-C (15). Inhibition or genetic knockdown of HDC ablated these tumorigenic effects by decreasing proliferation, lowering VEGF expression and diminishing tumor growth in vivo and in vitro (15).
Besides histamine deactivation or receptor interaction, other compounds have been found to both decrease cholangiocarcinoma growth and angiogenesis or metastatic potential. In a study using the peptide endothelial-1 or ET-1, the authors found that treatment with ET-1 decreased both in vitro and in vivo growth of cholangiocarcinoma growth (43). Further, the receptors of endothelin, ET-A and ET-B were both upregulated in tumors and cholangiocarcinoma cell lines compared to normal controls (43). This study is contradictory to other studies demonstrating that ET-1 is growth promoting, however interaction with ET-1 during cholangiocarcinoma appears to be regulated via upregulation of the receptors and inhibitors blocking these receptors contributed to tumor growth (43). Another neurosensory peptide that has been shown to effectively decrease cholangiocarcinoma growth and VEGF expression is the inhibitory neurotransmitter, gamma-aminobutyric acid (GABA) (44). In this study, it was demonstrated that GABA induces a decrease in tumor growth and VEGF-A and VEGF-C expression whilst increasing the apoptotic response of cholangiocarcinoma cells via inhibition of adenylate cyclase signaling (44).
As we've reviewed, the role of VEGF during angiogenesis is widely accepted although a study from Tang, et al. could not find any significant correlation between VEGF, Ang-1 and Ang- 2, expression and tumor size, capsule formation, infiltration of capsule, portal vein invasion, intrahepatic metastasis or CCC differentiation (45). Limitations of this study include the identification and distinction of which VEGF protein and receptor the authors examined along with the conclusion that, although VEGF was not altered, they observed a higher rate of angiogenesis in cholangiocarcinoma samples compared to control (45). Studies involving VEGF-regulation of angiogenesis remain to be elusive, however evidence strongly suggests that VEGF is a key mediator of angiogenesis during tumor formation and growth.
microRNA regulation of VEGF
Advancements in science and research have brought our understanding of cancer progression and tumor angiogenesis to new heights over the years and most recently the role or microRNAs has been at the forefront. It has been recognized that microRNAs might participate in the process of angiogenesis by activating angiogenic factors and the angiogenic switch (46). microRNAs are small, non-coding RNAs that are believed to influence many cellular activities including proliferation, differentiation and potentially in angiogenic activity (47). Here we will discuss briefly the regulation of VEGF by microRNAs in a broad sense and not specifically targeting cholangiocarcinoma. Although the majority of the studies are not specifically in cholangiocarcinoma, we believe that these studies are forthcoming and will be critical to development therapies for cholangiocarcinoma. A recent review has highlighted the potential importance of microRNAs and the regulation of the angiogenic switch (47). This review implicates several microRNAs including miR-126, miR-296, miR-132 and miR-92a as participating in the regulation of angiogenesis (47). miR-126 and its complement, miR-126* may promote the progression of cancer, which could involve the development of blood vessel growth and inflammation (48). In a study by Chamorro, et al. the authors found that specific microRNAs, miR-16 and miR- 424 may regulate angiogenic processes in endothelial cells (49). Specifically, they found that the expression of VEGFR2 and FGFR1 (induced by miR-16 or miR-424 overexpression) regulated VEGF and FGF signaling through these receptors. Regulation of VEGF and FGF signaling resulted in reduced cell migration and proliferation and further overexpression of miR-16 diminished the ability of ECs to form blood vessels in vivo, thus decreasing the success of angiogenesis (49). In human breast cancer, miR-126 expression was found to be downregulated and able to target VEGF-A. Overexpression of miR-126 decreased VEGF signaling activity suggesting that this miR regulates breast cancer tumorigenesis and angiogenesis (50). In pancreatic cancer (a close relative of cholangiocarcinoma (REF), overexpression of miR-20a reduced cell proliferation and invasion in two pancreatic cell lines suggesting that miR regulation could offer an alternative to current pancreatic cancer treatment (51). Collectively, these important studies lend another layer of both complication and potential regulation during tumor angiogenesis.
Clinical implications of VEGF inhibition
Because of the important role that VEGF plays during angiogenesis it has been targeted as a potential therapeutic target and clinical implications of VEGF inhibition may aide in the treatment of cholangiocarcinoma. A recent study has found that sorafenib, a multikinase inhibitor, which acts primarily through inhibition of VEGF and platelet-derived growth factor (PDGF) receptors, exhibited potent antitumor activity in hepatocellular cancer cells (52). Further, oral administration of sorafenib has been shown to prolong survival and decrease tumor growth in intrahepatic cholangiocarcinoma mouse models (53). Another study has shown that sorafenib sensitizes cholangiocarcinoma cells to undergo apoptosis and in an orthotopic syngeneic CCA model in rats, sorafenib significantly decreased tumor progression (54). These studies suggest that the VEGF inhibitor, sorafenib may offer a therapeutic advantage to patients suffering from cholangiocarcinoma.
The specific VEGF-R2/EGFR inhibitor, vandetanib (ZD6474, ZACTIMA) has also been found to be clinically relevant in blocking VEGF expression and decreasing angiogenesis and cholangiocarcinoma tumor growth. This drug is orally available and currently in Phase 1 clinical development for cancer treatment (55). Vandetanib treatment decreases both tumor growth in a cholangiocarcinoma xenograft mouse model and prolonged the time for the tumor to metastasize demonstrating its effectiveness as a potential option for therapy (56). In both gastric and colon cancer cells, vandetanib was also found to reduce soft-agar colony formation and in nude mice implanted with colon cancer cells, vandetanib diminished tumor growth in a dose-dependent manner (55). The antitumor activity of vandetanib in colon tumor xenografts is enhanced when combined with the drug paclitaxel, (a therapeutic cancer treatment also referred to as Taxol ®) and tumor regression was found in all animals after treatment with vandetanib plus paclitaxel, and this was accompanied by a significant inhibition of angiogenesis (55). A further study using colon cancer xenografts found that when in combination, the potent VEGF receptor inhibitor, vandentnib and cetuximab, an anti-EGFR blocking monoclonal antibody, a more significant tumor growth delay resulted with an increase in mice median overall survival as compared to single agent treatment (57). As stated, EGFR and VEGF play an important role in cholangiocarcinoma and other GI cancer growth. Enhanced cyclooxygenase-2 (COX-2) expression has been linked to cancer cell proliferation (including cholangiocarcinoma growth (36,58)), EGFR activation, VEGF secretion, and tumor-induced angiogenesis (59). Vandetanib (ZD6474) was combined with a COX-2 inhibitor, SC-236, and colon cancer growth was examined. In cancer xenografts treated with ZD6474 and/or SC-236 for 3 weeks, reversible tumor growth inhibition was found with each agent, whereas an increased prolonged inhibitory effect (lasting 3-5 weeks) resulted from the combination of the two agents (59). Further, 10-week treatment with ZD6474 plus SC-236 resulted in sustained tumor growth inhibition in all mice with tumor eradication in 3 out of 10 colon tumor-bearing mice (59). As cholangiocarcinoma is similar in morphology and progression to colon cancer (4), it is likely that these therapeutic agents will induce similar responses in cholangiocarcinoma cells and tumors. Taken together, these studies strongly suggest that inhibition of VEGF receptors (alone and in combination with other inhibitors) may be key to the eradication of cholangiocarcinoma or, at minimum, provide prolonged survival time in patients struggling with this disease.
Concluding remarks
As we have reviewed, cholangiocarcinoma tumors are in close proximity to their blood supply because they require the nutrients, oxygen, and waste removal functions that the vascular system provides for continued life and proliferation. These tumors are able to generate their own blood supply by increasing the expression of VEGF and VEGF receptors. This process allows cholangiocarcinoma to become invasive and metastatic. Regulation and inhibition of VEGF and VEGF receptors by hormones, peptides and microRNAs provides new therapeutic insights into treatment options for patients suffering from cholangiocarcinoma.
References
- Blechacz BR, Gores GJ. Cholangiocarcinoma. Clin Liver Dis 2008;12:131- 50, ix.[LinkOut]
- de Groen PC, Gores GJ, LaRusso NF, et al. Biliary tract cancers. N Engl J Med 1999;341:1368-78.[LinkOut]
- Francis H, Alpini G, DeMorrow S. Recent advances in the regulation of cholangiocarcinoma growth. Am J Physiol Gastrointest Liver Physiol 2010;299:G1-9.[LinkOut]
- Sirica AE. Cholangiocarcinoma: molecular targeting strategies for chemoprevention and therapy. Hepatology 2005;41:5-15.[LinkOut]
- Welzel TM, McGlynn KA, Hsing AW, et al. Impact of classification of hilar cholangiocarcinomas (Klatskin tumors) on the incidence of intra- and extrahepatic cholangiocarcinoma in the United States. J Natl Cancer Inst 2006;98:873-5.[LinkOut]
- Farley DR, Weaver AL, Nagorney DM. "Natural history" of unresected cholangiocarcinoma: patient outcome after noncurative intervention. Mayo Clin Proc 1995;70:425-9.[LinkOut]
- Patel T. Worldwide trends in mortality from biliary tract malignancies. BMC Cancer 2002;2:10.[LinkOut]
- Casavilla FA, Marsh JW, Iwatsuki S, et al. Hepatic resection and transplantation for peripheral cholangiocarcinoma. J Am Coll Surg 1997;185:429-36.[LinkOut]
- Lieser MJ, Barry MK, Rowland C, et al. Surgical management of intrahepatic cholangiocarcinoma: a 31-year experience. J Hepatobiliary Pancreat Surg 1998;5:41-7.[LinkOut]
- Madariaga JR, Iwatsuki S, Todo S, et al. Liver resection for hilar and peripheral cholangiocarcinomas: a study of 62 cases. Ann Surg 1998;227:70-9.[LinkOut]
- Ohtsuka M, Ito H, Kimura F, et al. Results of surgical treatment for intrahepatic cholangiocarcinoma and clinicopathological factors influencing survival. Br J Surg 2002;89:1525-31.[LinkOut]
- Stutes M, Tran S, DeMorrow S. Genetic and epigenetic changes associated with cholangiocarcinoma: from DNA methylation to microRNAs. World J Gastroenterol 2007;13:6465-9.[LinkOut]
- Meng F, Henson R, Lang M, et al. Involvement of human micro-RNA in growth and response to chemotherapy in human cholangiocarcinoma cell lines. Gastroenterology 2006;130:2113-29.[LinkOut]
- Xu LB, Liu C, Gao GQ, et al. Nerve growth factor-beta expression is associated with lymph node metastasis and nerve infiltration in human hilar cholangiocarcinoma. World J Surg 2010;34:1039-45.[LinkOut]
- Francis H, Demorrow S, Venter J, et al. Inhibition of histidine decarboxylase ablates the autocrine tumorigenic effects of histamine in human cholangiocarcinoma. Gut 2011. [Epub ahead of print][LinkOut]
- Catalano OA, Sahani DV, Forcione DG, et al. Biliary infections: spectrum of imaging findings and management. Radiographics 2009;29:2059-80.[LinkOut]
- Chapman RW. Risk factors for biliary tract carcinogenesis. Ann Oncol 1999;10:308-11.[LinkOut]
- Chen MF. Peripheral cholangiocarcinoma (cholangiocellular carcinoma): clinical features, diagnosis and treatment. J Gastroenterol Hepatol 1999;14:1144-9.[LinkOut]
- Gores GJ. Cholangiocarcinoma: current concepts and insights. Hepatology 2003;37:961-9.[LinkOut]
- Lipsett PA, Pitt HA, Colombani PM, et al. Choledochal cyst disease. A changing pattern of presentation. Ann Surg 1994;220:644-52.[LinkOut]
- Shaib YH, El-Serag HB, Nooka AK, et al. Risk factors for intrahepatic and extrahepatic cholangiocarcinoma: a hospital-based case-control study. Am J Gastroenterol 2007;102:1016-21.[LinkOut]
- Vasinca D, Pîrlog P. [The role of nurseries in ensuring the continuity of nursing care and the harmonious development of the child]. Viata Med Rev Inf Prof Stiint Cadrelor Medii Sanit 1986;34:193-4.[LinkOut]
- Meng F, Han Y, Staloch D, et al. The H4 histamine receptor agonist, clobenpropit, suppresses human cholangiocarcinoma progression by disruption of epithelial mesenchymal transition and tumor metastasis. Hepatology 2011;54:1718-28.[LinkOut]
- Leite de Oliveira R, Hamm A, Mazzone M. Growing tumor vessels: more than one way to skin a cat - implications for angiogenesis targeted cancer therapies. Mol Aspects Med 2011;32:71-87.[LinkOut]
- Alvaro D, Macarri G, Mancino MG, et al. Serum and biliary insulin-like growth factor I and vascular endothelial growth factor in determining the cause of obstructive cholestasis. Ann Intern Med 2007;147:451-9.[LinkOut]
- Lazaridis KN, Gores GJ. Cholangiocarcinoma. Gastroenterology 2005;128:1655-67.[LinkOut]
- Gaudio E, Franchitto A, Pannarale L, et al. Cholangiocytes and blood supply. World J Gastroenterol 2006;12:3546-52.[LinkOut]
- Gerhardt H, Golding M, Fruttiger M, et al. VEGF guides angiogenic sprouting utilizing endothelial tip cell filopodia. J Cell Biol 2003;161:1163- 77.[LinkOut]
- Shalaby F, Rossant J, Yamaguchi TP, et al. Failure of blood-island formation and vasculogenesis in Flk-1-deficient mice. Nature 1995;376:62-6.[LinkOut]
- Jain RK. Normalization of tumor vasculature: an emerging concept in antiangiogenic therapy. Science 2005;307:58-62.[LinkOut]
- Gialeli C, Theocharis AD, Karamanos NK. Roles of matrix metalloproteinases in cancer progression and their pharmacological targeting. FEBS J 2011;278:16-27.[LinkOut]
- Grassian AR, Coloff JL, Brugge JS. Extracellular Matrix Regulation of Metabolism and Implications for Tumorigenesis. Cold Spring Harb Symp Quant Biol 2011. [Epub ahead of print][LinkOut]
- Thelen A, Scholz A, Weichert W, et al. Tumor-associated angiogenesis and lymphangiogenesis correlate with progression of intrahepatic cholangiocarcinoma. Am J Gastroenterol 2010;105:1123-32.[LinkOut]
- Bolenz C, Fernández MI, Tilki D, et al. The role of lymphangiogenesis in lymphatic tumour spread of urological cancers. BJU Int 2009;104:592-7.[LinkOut]
- Miwa S, Soeda J, Miyagawa S. Interrelationship of platelet-derived endothelial cell growth factor, liver macrophages, and tumor microvessel density in patients with cholangiocellular carcinoma. Hepatogastroenterology 2005;52:1398-402.[LinkOut]
- Prakobwong S, Khoontawad J, Yongvanit P, et al. Curcumin decreases cholangiocarcinogenesis in hamsters by suppressing inflammationmediated molecular events related to multistep carcinogenesis. Int J Cancer 2011;129:88-100.[LinkOut]
- Mancino A, Mancino MG, Glaser SS, et al. Estrogens stimulate the proliferation of human cholangiocarcinoma by inducing the expression and secretion of vascular endothelial growth factor. Dig Liver Dis 2009;41:156- 63.[LinkOut]
- Takamura M, Yamagiwa S, Wakai T, et al. Loss of liver-intestine cadherin in human intrahepatic cholangiocarcinoma promotes angiogenesis by upregulating metal-responsive transcription factor-1 and placental growth factor. Int J Oncol 2010;36:245-54.[LinkOut]
- Gaudio E, Barbaro B, Alvaro D, et al. Administration of r-VEGF-A prevents hepatic artery ligation-induced bile duct damage in bile duct ligated rats. Am J Physiol Gastrointest Liver Physiol 2006;291:G307-17.[LinkOut]
- Gaudio E, Barbaro B, Alvaro D, et al. Vascular endothelial growth factor stimulates rat cholangiocyte proliferation via an autocrine mechanism. Gastroenterology 2006;130:1270-82.[LinkOut]
- Hicklin DJ, Ellis LM. Role of the vascular endothelial growth factor pathway in tumor growth and angiogenesis. J Clin Oncol 2005;23:1011-27.[LinkOut]
- Francis H, Onor i P, Gaudio E, et al. H3 histamine receptormediated activation of protein kinase Calpha inhibits the growth of cholangiocarcinoma in vitro and in vivo. Mol Cancer Res 2009;7:1704-13.[LinkOut]
- Fava G, Demorrow S, Gaudio E, et al . Endothe l in inhibi t s cholangiocarcinoma growth by a decrease in the vascular endothelial growth factor expression. Liver Int 2009;29:1031-42.[LinkOut]
- Fava G, Marucci L, Glaser S, et al. gamma-Aminobutyric acid inhibits cholangiocarcinoma growth by cyclic AMP-dependent regulation of the protein kinase A/extracellular signal-regulated kinase 1/2 pathway. Cancer Res 2005;65:11437-46.[LinkOut]
- Tang D, Nagano H, Yamamoto H, et al. Angiogenesis in cholangiocellular carcinoma: expression of vascular endothelial growth factor, angiopoietin-1/2, thrombospondin-1 and clinicopathological significance. Oncol Rep 2006;15:525-32.[LinkOut]
- Fish JE, Srivastava D. MicroRNAs: opening a new vein in angiogenesis research. Sci Signal 2009;2:pe1.[LinkOut]
- Anand S, Cheresh DA. MicroRNA-mediated regulation of the angiogenic switch. Curr Opin Hematol 2011;18:171-6.[LinkOut]
- Meister J, Schmidt MH. miR-126 and miR-126*: new players in cancer. Scientific World Journal 2010;10:2090-100.[LinkOut]
- Chamorro-Jorganes A, Araldi E, Penalva LO, et al. MicroRNA-16 and microRNA-424 regulate cell-autonomous angiogenic functions in endothelial cells via targeting vascular endothelial growth factor receptor-2 and fibroblast growth factor receptor-1. Arterioscler Thromb Vasc Biol 2011;31:2595-606.[LinkOut]
- Zhu N, Zhang D, Xie H, et al. Endothelial-specific intron-derived miR-126 is down-regulated in human breast cancer and targets both VEGFA and PIK3R2. Mol Cell Biochem 2011;351:157-64.[LinkOut]
- Yan H, Wu J, Liu W, et al. MicroRNA-20a overexpression inhibited proliferation and metastasis of pancreatic carcinoma cells. Hum Gene Ther 2010;21:1723-34.[LinkOut]
- Liu L, Cao Y, Chen C, et al. Sorafenib blocks the RAF/MEK/ERK pathway, inhibits tumor angiogenesis, and induces tumor cell apoptosis in hepatocellular carcinoma model PLC/PRF/5. Cancer Res 2006;66:11851- 8.[LinkOut]
- Sugiyama H, Onuki K, Ishige K, et al. Potent in vitro and in vivo antitumor activity of sorafenib against human intrahepatic cholangiocarcinoma cells. J Gastroenterol 2011;46:779-89.[LinkOut]
- Blechacz BR, Smoot RL, Bronk SF, et al. Sorafenib inhibits signal transducer and activator of transcription-3 signaling in cholangiocarcinoma cells by activating the phosphatase shatterproof 2. Hepatology 2009;50:1861-70.[LinkOut]
- Ciardiello F, Caputo R, Damiano V, et al. Antitumor effects of ZD6474, a small molecule vascular endothelial growth factor receptor tyrosine kinase inhibitor, with additional activity against epidermal growth factor receptor tyrosine kinase. Clin Cancer Res 2003;9:1546-56.[LinkOut]
- Yoshikawa D, Ojima H, Kokubu A, et al. Vandetanib (ZD6474), an inhibitor of VEGFR and EGFR signalling, as a novel molecular-targeted therapy against cholangiocarcinoma. Br J Cancer 2009;100:1257-66.[LinkOut]
- Morelli MP, Cascone T, Troiani T, et al. Anti-tumor activity of the combination of cetuximab, an anti-EGFR blocking monoclonal antibody and ZD6474, an inhibitor of VEGFR and EGFR tyrosine kinases. J Cell Physiol 2006;208:344-53.[LinkOut]
- Choi HJ, Kim HJ, Choi JH. Expression of c-erbB-2 and cyclooxygenase-2 in intrahepatic cholangiocarcinoma. Hepatogastroenterology 2009;56:606- 9.[LinkOut]
- Tuccillo C, Romano M, Troiani T, et al. Antitumor activity of ZD6474, a vascular endothelial growth factor-2 and epidermal growth factor receptor small molecule tyrosine kinase inhibitor, in combination with SC-236, a cyclooxygenase-2 inhibitor. Clin Cancer Res 2005;11:1268-76.[LinkOut]