Therapeutic actions of melatonin on gastrointestinal cancer development and progression
Melatonin
Melatonin is a natural compound with a multitude of physiological functions such as the regulation of the sleep cycle and the circadian rhythm (1,2). The primary sites of melatonin synthesis and secretion are the pineal gland as well as the gastrointestinal tract (e.g., small intestine and liver), the retinal pigment epithelium, lymphocytes and melanocytes in the skin (3-8). Although melatonin synthesis in the pineal gland is inhibited by exposure to light and activated during darkness, the release of melatonin in the gastrointestinal tract seems to be also dependent on food consumption (9,10). In some animal species, the levels of melatonin in the gastrointestinal tract exceed the blood levels by 10-100 times (11). In addition, the gastrointestinal tract contributes to the changes in melatonin concentration found in the peripheral blood (11,12). Melatonin affects the function of numerous cells and can be detected in membrane, cytoplasmic, nuclear, and mitochondrial compartments of several cell types (13). Also, melatonin plays important roles in anti-oxidative processes, and it has been shown to have immune-enhancing properties. Indeed, melatonin exerts its function by both autocrine and paracrine mechanisms, thus affecting the function of lymphatic tissues of the immune system, the gastrointestinal epithelia, and the smooth muscles of the digestive system (14). Also, melatonin improves T-helper cell response by the production of specific cytokines including interleukin-2 (IL-2), interleukin-6 (IL-6) interleukin-10 (IL-10), interleukin-12 (IL-12), and interferon-y (IFN-y) (15). T-Helper cells play a crucial role in the protection against malignancy. Indeed, studies have shown that melatonin prevents tumor initiation, promotion, and progression in a variety of tumors such melanoma, breast, prostate, ovarian, hepatocellular, biliary and colorectal cancer (16-25).
Gastrointestinal cancers
Pancreatic cancer is the third most common malignancy and the fifth foremost cause of cancer-associated death among gastrointestinal cancers (26-29). Pancreatic tumors are often resistant to chemotherapy and radiotherapy and resistant to apoptosis (30,31). Approximately, 80-95% of these tumors have mutations in the KRAS2 gene, and 85-98% of these tumors show mutations, deletions, or hypermethylation in the CDKN2 gene (26,32-34).
Though the incidence of gastric cancer is decreasing steadily around the world, it still remains a serious health problem since is the second most common type of fatal cancer (35-37). Extensive studies of potential therapeutic actions for gastric cancer have made progress, but the chance of success for patients with advanced gastric cancer is not favorable.
Colorectal cancer is one of the leading types of cancers of the gastrointestinal tract (38,39), and its prevalence has been predicted to increase significantly by the year 2020 (40). It has been estimated to increase from approximately 0.36% to 0.46% between 2000 and 2020 (40). Furthermore, it has been suggested that colorectal cancer prevalence will increase much more rapidly than the population in the United States (40).
Hepatocellular carcinoma is the fifth most common strain of cancer in the world with more that half a million of new cases every year (41-44). It has become a very serious health problem around the world because of its relation to infection with both hepatitis B and C viruses (45-47). Liver transplantation and chemotherapy are crucial therapeutic strategies utilized in the treatment of hepatocellular carcinoma.
Gastrointestinal cancer has taken precedence in oncological research seeking to improve therapeutic treatments, and recently, a major approach to this field has been to examine the role of melatonin in the tumorigenesis of gastrointestinal cancers.
Melatonin biosynthesis
Melatonin is synthesized in the pineal gland, and released into the serum in a circadian pattern, corresponding to light and dark cycles (4). After leaving the pineal gland, melatonin enters the blood stream as an endocrine compound, and regulates biological rhythms such as the sleep-wake cycle. Recent studies have shown that melatonin is synthesized in a number of tissues/organs including in the eye, lymphocytes, mast cells and the gastrointestinal tract (3-7). Melatonin released by these peripheral tissues is not released into the blood in excessive amounts (as opposed to the amount produced in the pineal gland) but rather it acts locally as an autocrine and/or paracrine signals (48,49). The exposure to light and/or dark, which regulates the release of melatonin by the pineal gland, is received in the suprachiasmatic nuclei via retinal photosensitive ganglionic cells (13). Superior cervical ganglion (SCG) postganglionic neurons produce fibers that stimulate the pineal gland and release norepinephrine, thus regulating melatonin synthesis. Norepinephrine released during the night binds to β-adrenergic receptors on pinealocytes, thus activating adenylyl cyclase/cAMP signaling that stimulates the synthesis of melatonin (50,51). During the daytime, the suprachiasmatic nucleus (which regulates the circadian oscillation in the brain) inhibits the release of norepinephrine, suppressing the production of melatonin (52).
Melatonin synthesis starts from the amino acid tryptophan, which is then converted into 5-hydroxytryptophan. At that point, it becomes serotonin, which is then acetylated to form N-acetylserotonin. This process happens by the action of the enzyme, arylalkylamine-N-acetyltransferase. The acetylated serotonin is then converted into melatonin by hydroxyindole-O-methyltransferase, which is the rate-determining enzyme (slow-step) in the synthesis of melatonin (53).
Production of melatonin appears to decline gradually with aging as elderly individuals have been shown to have low levels of melatonin (54,55). However, it is possible that other factors besides aging regulate the change in melatonin levels in elderly people (54). Other factors associated with age such as chronic diseases, depressed mood, physical disabilities, poor perceived health may contribute to sleep disturbances that would affect the circadian rhythm of older people contributing to the reduction in melatonin levels (54).
Melatonin in the lower gut
Melatonin levels in the gastrointestinal tract are unrelated to melatonin levels synthesized in the pineal gland. These proposed findings are supported by the fact that pinealectomy in rats have no influence on the levels of melatonin in the gastrointestinal tract (56). Also, the concentrations of melatonin in the gastrointestinal tract decrease with aging. Indeed, studies have shown that the melatonin levels in the mucosa of the ileum and distal colon are higher in older compared to younger mice (57). Circulating melatonin during the daytime may originate from the gastrointestinal tract (57). These variations in melatonin levels also suggest that melatonin regulates the digestion and control of food consumption.
Even though several animal studies show protective effects of melatonin in colitis models, the therapeutic role of melatonin in inflammatory bowel disease (IBD) is still unclear (13). Several research groups have tested the effects of the self-administration of melatonin in IBD. It has been presented that the self-administered use of melatonin as a self-directed treatment for jet lag on international flights, the patient observed that his ulcerative colitis (UC) symptoms were virtually absent (58,59). Meanwhile, other studies showed melatonin exacerbated symptoms associated with UC or Crohn’s disease (60,61). Further studies, especially clinical trials should be performed to evaluate a possible beneficial or detrimental effect of melatonin in IBD despite those basic researches strongly suggest beneficial effects.
Melatonin metabolism
Melatonin is primarily metabolized in the liver, where it is hydroxylated in the sixth carbon position by cytochrome P450 mono-oxygenases. Then, it is conjugated to sulfate and released as 6-sulfatoxymelatonin (62).
Melatonin is metabolized in all cells without the use of enzymes, and it can also be metabolized extracellularly with oxidants and free radicals. Through these steps melatonin is converted into cyclic-3-hydroxy melatonin when it acquires two hydroxyl radicals. This process is referred to as the kynurenine pathway of melatonin metabolism, in which enzymatic reactions, pseudo enzymatic reaction involving oxoferryl-hemoglobin, photo catalytic reactions, reactions with oxygen free radicals, and ozonolysis take place. During the conversion process of melatonin to N1-acetyl-N2-formyl-5-methoxykynuramine (AFMK) as many as four radicals can be consumed. It has been suggested that AFMK is the primary metabolite of melatonin (63).
Melatonin receptors
Some of the effects of melatonin depend on the activation of membrane receptors, which are also expressed in the gastrointestinal tract including the ileum, colon and the liver (13,16,64,65). These receptors are named melatonin-1 receptor (MT1), MT2, and MT3. MT1 and MT2 are part of the G-protein coupled receptor (GPCR) family, and they share a common seven-transmembrane structure. These two receptors activate distinct intracellular signaling pathways, some of which mediate adenylate cyclase inhibition (64) and phospholipase C beta activation (66,67). The MT2 receptor couples to several signaling pathways including phosphoinositol production, the inhibition of guanylate cyclase pathway as well as the inhibition of adenylate cyclase. MT3, on the other hand, binds to the enzyme, quinonereductase 2 (QR2). Activation of this enzyme-substrate complex by melatonin suggests that melatonin protect against oxidative stress due to its antioxidative properties (13).
In a study performed in rat pancreas, stomach, colon, and duodenum, MT2 immunoreactivity was found in the muscularis mucosae and the circular and longitudinal muscle layers of the gastrointestinal tract (68). These findings suggest that the MT2 receptors may regulate intestinal motility. Melatonin receptors may also mediate the effects of melatonin on the efficacy of the immune system.
Melatonin and the immune system
Melatonin can also increase natural killer cell activity and Th2 cell-mediated immune responses (69,70). Also, melatonin has been shown to protect human and murine CD4+ T cells from apoptosis by inhibiting CD95 ligand mRNA and protein up-regulation in response to TCR/CD3 stimulation (71). Melatonin affects T cell tolerance by a IL-2-dependent pathway, but the vast majority of the effects of melatonin on lymphocyte function is mediated by MT1 receptors (72).
Increased numbers of CD4+ CD25+ regulatory T cells (Tregs) have been demonstrated in the tumor of patients and animal models with gastric cancer. A recent has evaluated the role of Tregs in melatonin inhibition of gastric cancer growth both in vivo and in vitro (73). This study has also shown that melatonin decreased the number of Tregs and Forkhead box p3 (Foxp3) expression in tumor tissue. The study suggested that melatonin inhibition of gastric cancer could be due to down-regulation of CD4+ CD25+ Tregs and Foxp3 expression in tumor tissue (73).
Melatonin and colorectal cancer
A recent study has demonstrated the presence of melatonin binding sites in the mucosa and the submucosa of the human colon (74). Specifically, in this study it was demonstrated (by radioimmunoassay) that melatonin concentration in control patient tissues was 467±99 pg/g, whereas daytime melatonin levels in colorectal cancer patients were 3,147±87.8 pg/g tissue (74).
It was also found that increased colonic crypt cell proliferation had occurred in rats, indicating that melatonin pathways were involved in tumorigenesis in the colon (75). Another study suggested that small bowel crypt cell hyperplasia occurred after the pinealectomy, but the mechanism by which this occurred was not evaluated (13). In a recent study, it has been shown that melatonin regulates preneoplastic patterns caused by constant light exposure in the colon (76). The proposed mechanism by which melatonin controls colon cancer involves the inhibition of tumor angiogenesis, maintenance of the intracellular level of glutathione, and modulation of mitotic and apoptotic processes.
Other factors affecting tumor development in relation to melatonin include the modulation of estrogen receptors, regulation of cell cycle, expression of growth factors and antioxidants, and increased gap junctions (77,78). Melatonin has also been shown to alter the oxidative properties and to reduce the rate of production of nitric oxide in cultured colon cancer cells (79). The clinical trials that have been conducted using melatonin in relation to colorectal cancer are few and not of great quality. These studies have not yielded data to confirm the efficacy of melatonin for colorectal cancer. Controlled clinical trials are still needed in order to establish melatonin’s role in cancer treatment more concretely.
Melatonin in hepatocellular carcinoma and cholangiocarcinoma
A recent study has demonstrated that melatonin inhibits the growth of rat hepatoma 7288CTC cells by a receptor-mediated inhibition of tumor linoleic acid uptake and its metabolism to 13-hydroxyoctadecadienoic acid (13-HODE), which is the mitogenic signal for linoleic acid-dependent tumor growth (80). Melatonin inhibition of the growth of the growth of rat hepatoma 7288CTC cells was also associated with reduced cAMP levels, and phosphorylation of ERK 1/2 (80).
In a recent study which was intended to observe and evaluate the effects of anticarcinogenic functions of melatonin, N-nitrosodiethylamine (NDEA), which is a carcinogen that induces liver cancer, was used to inject Wistar male rats (81). This was followed by weekly injections of carbon tetrachloride or vehicle for six weeks. Melatonin or its vehicle was administered two hours before lights out on a daily basis for 20 weeks. After dissection, the results showed that the NDEA had stunted body weight gain and produced sizable liver tumors (81). In addition, NDEA increased levels of alanine transaminase, plasma aspartate transaminase, and α-fetoprotein. Administration of melatonin proved to have significantly hindered tumor development, presumably by way of its antioxidant effects, both direct and indirect.
In a clinical trial with 35 patients having advanced tumors of the digestive tract, a treatment regimen utilizing low-dose IL-2 and melatonin was tested (82). Fourteen patients had colorectal cancer, eight had gastric cancer, six had hepatic carcinoma, and seven had pancreatic adenocarcinoma. Complete response was achieved in two of the patients, one of which had gastric cancer and the other hepatic carcinoma. An additional six patients showed tumor regression.
In another study, patients with advanced primary hepatocellular carcinoma were treated with transcatheter arterial chemoembolization (TACE) alone, or simultaneously with melatonin (83). The efficacy of the TACE alone was 16 percent, whereas the efficacy of TACE with melatonin was 28 percent. The survival rate of half, one, and two years in the TACE group was 82 percent, 54 percent, and 26 percent (83). The survival rate of the TACE and melatonin group was 100 percent, 68 percent, and 40 percent (83).
Cholangiocarcinoma is a cancer that arises from the malignant transformation of bile duct epithelial cells (i.e., cholangiocytes) (84-86). The occurrence of cholangiocarcinoma has been on the rise for the past decades (87-89). Cholangiocarcinoma is often anti-symptomatic until it reaches an advanced stage in its development, in which it becomes characterized by obstructive symptoms (89). Cholangiocarcinoma is resistant to conventional forms of chemotherapy, with surgical resection being the only effective therapy (89). We have recently shown that the enzymes [serotonin N-acetyltransferase or (AANAT), and acetylserotonin O-methyltransferase (ASMT)] regulating melatonin synthesis as well melatonin secretion are downregulated in cholangiocarcinoma lines and human biopsy samples compared to normal controls (16). Conversely, the expression of the melatonin receptors, MT1 and MT2, increased in CCA lines and biopsy samples compared to controls (16). Also, melatonin decreased the growth of human cholangiocarcinoma lines implanted into nude mice by increased AANAT, ASMT expression and melatonin synthesis and decreased MT1/MT2 expression (16) (Figure 1A,B). We also observed increased necrosis in sections from melatonin-treated mice compared with control mice (Figure 1A,B). There was a decrease in cholangiocarcinoma proliferation (by proliferating cell nuclear antigen (PCNA)) and an increase in biliary apoptosis (by cleaved caspase-3) in tumor sections from melatonin-treated mice compared to control mice (Figure 1A,B). Overexpression of AANAT was also shown to inhibit cholangiocarcinoma growth (16). We concluded that there is dysregulation of the AANAT/ASMT/melatoninmelatonin receptor axis in cholangiocarcinoma that decreased melatonin secretion, thus enhancing cholangiocarcinoma growth.
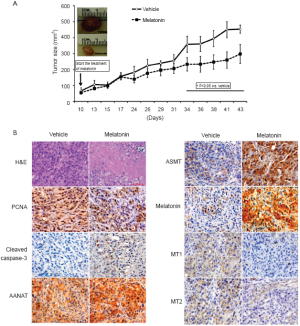
Melatonin as an adjuvant therapy for hepatocellular carcinoma
One previous study examined the cytostatic efficiency of doxorubicin applied together with melatonin to rats with transplantable Morris hepatoma (90). It was demonstrated that administration of melatonin together with doxorubicin decreased the extent of tumour necrosis and the apoptotic tumour cells but, on the other hand, decreased the number of apoptotic cardiomyocytes. Therefore, melatonin weakens the cytotoxic activity of doxorubicin by the decreased proportions of necrotic and apoptotic cells of transplantable Morris hepatoma. Melatonin protects also cardiomyocytes by decreasing doxorubicin-induced apoptosis in the cells.
In a recent study aimed to evaluate the growth-inhibiting and apoptosis-inducing effects of melatonin alone or in combination with chemotherapeutic drugs (e.g., Doxorubicin), melatonin was shown to inhibit the growth of HepG2 and Bel-7402 cells in a dose-dependent manner (91). The combination of the two treatments increased the inhibitory effect and induction of hepatocyte apoptosis compared to the individual treatments. In summary, melatonin and Doxorubicin synergistically affect the growth of the human hepatoma cell lines, HepG2 and Bel-7402 by down-regulation of Bcl-2 and up-regulation of Bax (91).
Melatonin in pancreatic carcinoma cells
Heat shock proteins are chaperones that regulate protein folding and degradation. Recent studies suggest that heat shock protein 27 (HSP27) regulate the promotion of tumor growth and the development of resistance to chemotherapy and radiotherapy (92).
In this contest, a recent study has shown that melatonin affects HSP27 protein expression in human pancreatic carcinoma cells (PANC-1) (93). Specifically, treatment with melatonin increased not only the cytoplasmic protein level, but also the nuclear phosphorylated HSP27 protein level in the PANC-1 cells in comparison to control cells. The authors suggested that melatonin stimulates the production and phosphorylation of HSP27 in PANC-1 and possibly inhibits apoptosis in these cells.
Conclusions
The antiproliferative and immunomodulatory effects of melatonin have been demonstrated in a multitude of cancers including those of the gastrointestinal tract. Melatonin has shown some success in the treatment of advanced hepatocellular carcinoma in combination with doxorubicin, through the inducement of cellular apoptosis, and the inhibition of cell proliferation (Figure 2). At the same time, melatonin has been shown to successfully enhance the immunomodulatory effects of IL-2 therapy (Figure 2). In cholangiocarcinoma, melatonin inhibited carcinogenesis in vivo and in vitro by both autocrine/paracrine mechanisms. The antioxidative effects of melatonin have been shown through further observation of melatonin receptors in the inhibition of several signaling pathways in colorectal and liver cancer. In summary, recent developments in the role and pathology of melatonin in cancers of the gastrointestinal tract have contributed to the improvement of therapeutic treatments for these cancers. The autocrine/paracrine modulation (e.g., by specific agonists/antagonists and/or exposure to dark/light) of melatonin synthesis by changes in the enzymes (AANAT and ASMT) regulating the synthesis of this chemical may be an important therapeutic tool for the management of a number of cancers.
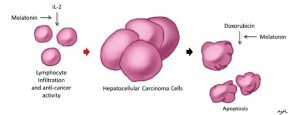
Acknowledgements
This work was supported by the Dr. Nicholas C. Hightower Centennial Chair of Gastroenterology from Scott & White Hospital, a VA Research Career Scientist Award, and the NIH grants DK58411 and DK062975 to both Drs. Alpini and Glaser and the NIH grant DK081442 to Dr. Glaser.
Disclosure : The authors declare no conflict of interest.
References
- Kitamura S, Hida A, Enomoto M, et al. Intrinsic Circadian Period of Sighted Patients with Circadian Rhythm Sleep Disorder, Free-Running Type. Biol Psychiatry 2013;73:63-9.
- Johnson N, Jain G, Sandberg L, et al. Modeling the effect of exogenous melatonin on the sleep-wake switch. Biomed Sci Instrum 2012;48:202-9.
- Bubenik GA. Gastrointestinal melatonin: localization, function, and clinical relevance. Dig Dis Sci 2002;47:2336-48.
- Reiter RJ. Pineal melatonin: cell biology of its synthesis and of its physiological interactions. Endocr Rev 1991;12:151-80.
- Agorastos A, Huber CG. The role of melatonin in glaucoma: implications concerning pathophysiological relevance and therapeutic potential. J Pineal Res 2011;50:1-7.
- Carrillo-Vico A, Calvo JR, Abreu P, et al. Evidence of melatonin synthesis by human lymphocytes and its physiological significance: possible role as intracrine, autocrine, and/or paracrine substance. FASEB J 2004;18:537-9.
- Slominski A, Tobin DJ, Zmijewski MA, et al. Melatonin in the skin: synthesis, metabolism and functions. Trends Endocrinol Metab 2008;19:17-24.
- Chojnacki C, Walecka-Kapica E, Klupińska G, et al. Serotonin and melatonin secretion and metabolism in patients with hepatic cirrhosis. Pol Arch Med Wewn 2012;122:392-7.
- Konturek PC, Brzozowski T, Konturek SJ. Gut clock: implication of circadian rhythms in the gastrointestinal tract. J Physiol Pharmacol 2011;62:139-50.
- Konturek PC, Brzozowski T, Konturek SJ. Stress and the gut: pathophysiology, clinical consequences, diagnostic approach and treatment options. J Physiol Pharmacol 2011;62:591-9.
- Bubenik GA. Thirty four years since the discovery of gastrointestinal melatonin. J Physiol Pharmacol 2008;59:33-51.
- Stebelová K, Herichová I, Zeman M. Diabetes induces changes in melatonin concentrations in peripheral tissues of rat. Neuro Endocrinol Lett 2007;28:159-65.
- Chen CQ, Fichna J, Bashashati M, et al. Distribution, function and physiological role of melatonin in the lower gut. World J Gastroenterol 2011;17:3888-98.
- Iwasaki S, Nakazawa K, Sakai J, et al. Melatonin as a local regulator of human placental function. J Pineal Res 2005;39:261-5.
- Srinivasan V, Pandi-Perumal SR, Brzezinski A, et al. Melatonin, immune function and cancer. Recent Pat Endocr Metab Immune Drug Discov 2011;5:109-23.
- Han Y, Demorrow S, Invernizzi P, et al. Melatonin exerts by an autocrine loop antiproliferative effects in cholangiocarcinoma: its synthesis is reduced favoring cholangiocarcinoma growth. Am J Physiol Gastrointest Liver Physiol 2011;301:G623-33.
- Lissoni P, Paolorossi F, Tancini G, et al. A phase II study of tamoxifen plus melatonin in metastatic solid tumour patients. Br J Cancer 1996;74:1466-8.
- Margheri M, Pacini N, Tani A, et al. Combined effects of melatonin and all-trans retinoic acid and somatostatin on breast cancer cell proliferation and death: molecular basis for the anticancer effect of these molecules. Eur J Pharmacol 2012;681:34-43.
- Slominski A, Pruski D. Melatonin inhibits proliferation and melanogenesis in rodent melanoma cells. Exp Cell Res 1993;206:189-94.
- Srinivasan V, Spence DW, Pandi-Perumal SR, et al. Therapeutic actions of melatonin in cancer: possible mechanisms. Integr Cancer Ther 2008;7:189-203.
- Sainz RM, Mayo JC, Tan DX, et al. Melatonin reduces prostate cancer cell growth leading to neuroendocrine differentiation via a receptor and PKA independent mechanism. Prostate 2005;63:29-43.
- Futagami M, Sato S, Sakamoto T, et al. Effects of melatonin on the proliferation and cis-diamminedichloroplatinum (CDDP) sensitivity of cultured human ovarian cancer cells. Gynecol Oncol 2001;82:544-9.
- Carbajo-Pescador S, García-Palomo A, Martín-Renedo J, et al. Melatonin modulation of intracellular signaling pathways in hepatocarcinoma HepG2 cell line: role of the MT1 receptor. J Pineal Res 2011;51:463-71.
- Motilva V, García-Mauriño S, Talero E, et al. New paradigms in chronic intestinal inflammation and colon cancer: role of melatonin. J Pineal Res 2011;51:44-60.
- Bubenik GA. Localization, physiological significance and possible clinical implication of gastrointestinal melatonin. Biol Signals Recept 2001;10:350-66.
- Jiang PH, Motoo Y, Sawabu N, et al. Effect of gemcitabine on the expression of apoptosis-related genes in human pancreatic cancer cells. World J Gastroenterol 2006;12:1597-602.
- Reichert M, Rustgi AK. Pancreatic ductal cells in development, regeneration, and neoplasia. J Clin Invest 2011;121:4572-8.
- Takao S, Ding Q, Matsubara S. Pancreatic cancer stem cells: regulatory networks in the tumor microenvironment and targeted therapy. J Hepatobiliary Pancreat Sci 2012;19:614-20.
- Kang R, Tang D. Autophagy in pancreatic cancer pathogenesis and treatment. Am J Cancer Res 2012;2:383-96.
- Wang F, Kumar P. The role of radiotherapy in management of pancreatic cancer. J Gastrointest Oncol 2011;2:157-67.
- Sato T, Kita K, Sugaya S, et al. Extracellular Release of Annexin II From Pancreatic Cancer Cells and Resistance to Anticancer Drug-Induced Apoptosis by Supplementation of Recombinant Annexin II. Pancreas 2012;41:1247-54.
- Shi G, Direnzo D, Qu C, et al. Maintenance of acinar cell organization is critical to preventing Kras-induced acinar-ductal metaplasia. Oncogene 2012. [Epub ahead of print].
- Ling J, Kang Y, Zhao R, et al. KrasG12D-induced IKK2/β/NF-κB activation by IL-1α and p62 feedforward loops is required for development of pancreatic ductal adenocarcinoma. Cancer Cell 2012;21:105-20.
- Hustinx SR, Leoni LM, Yeo CJ, et al. Concordant loss of MTAP and p16/CDKN2A expression in pancreatic intraepithelial neoplasia: evidence of homozygous deletion in a noninvasive precursor lesion. Mod Pathol 2005;18:959-63.
- Ebert MP, Schandl L, Malfertheiner P. Helicobacter pylori infection and molecular changes in gastric carcinogenesis. J Gastroenterol 2002;37:45-9.
- Oue N, Aung PP, Mitani Y, et al. Genes involved in invasion and metastasis of gastric cancer identified by array-based hybridization and serial analysis of gene expression. Oncology 2005;69:17-22.
- Koushi K, Korenaga D, Edagawa A, et al. Results of Laparoscopic Gastrectomy for Early Gastric Cancer in High-Risk Patients - Estimation of Surgical Risk of Gastrectomy. Hepatogastroenterology 2012. [Epub ahead of print].
- Dyson JK, Rutter MD. Colorectal cancer in inflammatory bowel disease: What is the real magnitude of the risk? World J Gastroenterol 2012;18:3839-48.
- Lofano K, Principi M, Scavo MP, et al. Dietary Lifestyle and Colorectal Cancer Onset, Recurrence, and Survival: Myth or Reality? J Gastrointest Cancer 2012. [Epub ahead of print].
- Mariotto AB, Yabroff KR, Feuer EJ, et al. Projecting the number of patients with colorectal carcinoma by phases of care in the US: 2000-2020. Cancer Causes Control 2006;17:1215-26.
- El-Serag HB, Rudolph KL. Hepatocellular carcinoma: epidemiology and molecular carcinogenesis. Gastroenterology 2007;132:2557-76.
- Hoshida Y, Fuchs BC, Tanabe KK. Prevention of hepatocellular carcinoma: potential targets, experimental models, and clinical challenges. Curr Cancer Drug Targets 2012;12:1129-59.
- Bishayee A, Thoppil RJ, Waghray A, et al. Dietary Phytochemicals in the Chemoprevention and Treatment of Hepatocellular Carcinoma: In Vivo Evidence, Molecular Targets, and Clinical Relevance. Curr Cancer Drug Targets 2012;12:1191-232.
- Braconi C, Patel T. Non-Coding RNAs as Therapeutic Targets in Hepatocellular Cancer. Curr Cancer Drug Targets 2012;12:1073-80.
- Melloul E, Lesurtel M, Carr BI, et al. Developments in liver transplantation for hepatocellular carcinoma. Semin Oncol 2012;39:510-21.
- Kwak MS, Lee DH, Cho Y, et al. Association of Polymorphism in Pri-microRNAs-371-372-373 with the Occurrence of Hepatocellular Carcinoma in Hepatitis B Virus Infected Patients. PLoS One 2012;7:e41983.
- Kuske L, Mensen A, Mã Llhaupt B, et al. Characteristics of patients with chronic hepatitis C who develop hepatocellular carcinoma. Swiss Med Wkly 2012;142:w13651.
- Reiter RJ. Mechanisms of cancer inhibition by melatonin. J Pineal Res 2004;37:213-4.
- Pandi-Perumal SR, Srinivasan V, Maestroni GJ, et al. Melatonin: Nature’s most versatile biological signal? FEBS J 2006;273:2813-38.
- Sugden LA, Sugden D, Klein DC. Alpha 1-adrenoceptor activation elevates cytosolic calcium in rat pinealocytes by increasing net influx. J Biol Chem 1987;262:741-5.
- Schomerus C, Laedtke E, Korf HW. Calcium responses of isolated, immunocytochemically identified rat pinealocytes to noradrenergic, cholinergic and vasopressinergic stimulations. Neurochem Int 1995;27:163-75.
- Moore RY, Danchenko RL. Paraventricular-subparaventricular hypothalamic lesions selectively affect circadian function. Chronobiol Int 2002;19:345-60.
- Liu T, Borjigin J. N-acetyltransferase is not the rate-limiting enzyme of melatonin synthesis at night. J Pineal Res 2005;39:91-6.
- Mishima K, Okawa M, Shimizu T, et al. Diminished melatonin secretion in the elderly caused by insufficient environmental illumination. J Clin Endocrinol Metab 2001;86:129-34.
- Pandi-Perumal SR, Bahammam AS, Brown GM, et al. Melatonin Antioxidative Defense: Therapeutical Implications for Aging and Neurodegenerative Processes. Neurotox Res 2012. [Epub ahead of print].
- Bubenik GA, Brown GM. Pinealectomy reduces melatonin levels in the serum but not in the gastrointestinal tract of rats. Biol Signals 1997;6:40-4.
- Bertrand PP, Bertrand RL, Camello PJ, et al. Simultaneous measurement of serotonin and melatonin from the intestine of old mice: the effects of daily melatonin supplementation. J Pineal Res 2010;49:23-34.
- Jan JE, Freeman RD. Re: Mann--melatonin for ulcerative colitis? Am J Gastroenterol 2003;98:1446.
- Mann S. Melatonin for ulcerative colitis? Am J Gastroenterol 2003;98:232-3.
- Maldonado MD, Calvo JR. Melatonin usage in ulcerative colitis: a case report. J Pineal Res 2008;45:339-40.
- Calvo JR, Guerrero JM, Osuna C, et al. Melatonin triggers Crohn’s disease symptoms. J Pineal Res 2002;32:277-8.
- Claustrat B, Brun J, Chazot G. The basic physiology and pathophysiology of melatonin. Sleep Med Rev 2005;9:11-24.
- Hardeland R. Antioxidative protection by melatonin: multiplicity of mechanisms from radical detoxification to radical avoidance. Endocrine 2005;27:119-30.
- Renzi A, Glaser S, Demorrow S, et al. Melatonin inhibits cholangiocyte hyperplasia in cholestatic rats by interaction with MT1 but not MT2 melatonin receptors. Am J Physiol Gastrointest Liver Physiol 2011;301:G634-43.
- León J, Casado J, Carazo A, et al. Gender-related invasion differences associated with mRNA expression levels of melatonin membrane receptors in colorectal cancer. Mol Carcinog 2012;51:608-18.
- von Gall C, Stehle JH, Weaver DR. Mammalian melatonin receptors: molecular biology and signal transduction. Cell Tissue Res 2002;309:151-62.
- Witt-Enderby PA, Bennett J, Jarzynka MJ, et al. Melatonin receptors and their regulation: biochemical and structural mechanisms. Life Sci 2003;72:2183-98.
- Stebelová K, Anttila K, Mänttäri S, et al. Immunohistochemical definition of MT(2) receptors and melatonin in the gastrointestinal tissues of rat. Acta Histochem 2010;112:26-33.
- Raghavendra V, Singh V, Kulkarni SK, et al. Melatonin enhances Th2 cell mediated immune responses: lack of sensitivity to reversal by naltrexone or benzodiazepine receptor antagonists. Mol Cell Biochem 2001;221:57-62.
- Shaji AV, Kulkarni SK, Agrewala JN. Regulation of secretion of IL-4 and IgG1 isotype by melatonin-stimulated ovalbumin-specific T cells. Clin Exp Immunol 1998;111:181-5.
- Pedrosa AM, Weinlich R, Mognol GP, et al. Melatonin protects CD4+ T cells from activation-induced cell death by blocking NFAT-mediated CD95 ligand upregulation. J Immunol 2010;184:3487-94.
- Sánchez-Hidalgo M, Lee M, de la Lastra CA, et al. Melatonin inhibits cell proliferation and induces caspase activation and apoptosis in human malignant lymphoid cell lines. J Pineal Res 2012;53:366-73.
- Liu H, Xu L, Wei JE, et al. Role of CD4+ CD25+ regulatory T cells in melatonin-mediated inhibition of murine gastric cancer cell growth in vivo and in vitro. Anat Rec (Hoboken) 2011;294:781-8.
- Poon AM, Mak AS, Luk HT. Melatonin and 2[125I]iodomelatonin binding sites in the human colon. Endocr Res 1996;22:77-94.
- Dalio MB, Haikel Júnior LF, Dalio RB, et al. A study of the effects of pinealectomy on intestinal cell proliferation in infant newborn rats. Acta Cir Bras 2006;21:16-20.
- Callaghan BD. The long-term effect of pinealectomy on the crypts of the rat gastrointestinal tract. J Pineal Res 1995;18:191-6.
- Panzer A, Viljoen M. The validity of melatonin as an oncostatic agent. J Pineal Res 1997;22:184-202.
- Wenzel U, Nickel A, Daniel H. Melatonin potentiates flavone-induced apoptosis in human colon cancer cells by increasing the level of glycolytic end products. Int J Cancer 2005;116:236-42.
- García-Navarro A, González-Puga C, Escames G, et al. Cellular mechanisms involved in the melatonin inhibition of HT-29 human colon cancer cell proliferation in culture. J Pineal Res 2007;43:195-205.
- Blask DE, Dauchy RT, Sauer LA, et al. Melatonin uptake and growth prevention in rat hepatoma 7288CTC in response to dietary melatonin: melatonin receptor-mediated inhibition of tumor linoleic acid metabolism to the growth signaling molecule 13-hydroxyoctadecadienoic acid and the potential role of phytomelatonin. Carcinogenesis 2004;25:951-60.
- Subramanian P, Mirunalini S, Dakshayani KB, et al. Prevention by melatonin of hepatocarcinogenesis in rats injected with N-nitrosodiethylamine. J Pineal Res 2007;43:305-12.
- Lissoni P, Barni S, Tancini G, et al. Immunotherapy with subcutaneous low-dose interleukin-2 and the pineal indole melatonin as a new effective therapy in advanced cancers of the digestive tract. Br J Cancer 1993;67:1404-7.
- Yan JJ, Shen F, Wang K, et al. Patients with advanced primary hepatocellular carcinoma treated by melatonin and transcatheter arterial chemoembolization: a prospective study. Hepatobiliary Pancreat Dis Int 2002;1:183-6.
- Sirica AE. The role of cancer-associated myofibroblasts in intrahepatic cholangiocarcinoma. Nat Rev Gastroenterol Hepatol 2011;9:44-54.
- Johnson C, Han Y, Hughart N, et al. Interleukin-6 and its receptor, key players in hepatobiliary inflammation and cancer. Transl Gastrointest Cancer 2012;1:58-70.
- Francis H, DeMorrow S, Venter J, et al. Inhibition of histidine decarboxylase ablates the autocrine tumorigenic effects of histamine in human cholangiocarcinoma. Gut 2012;61:753-64.
- Landis SH, Murray T, Bolden S, et al. Cancer statistics, 1998. CA Cancer J Clin 1998;48:6-29.
- Blechacz B, Komuta M, Roskams T, et al. Clinical diagnosis and staging of cholangiocarcinoma. Nat Rev Gastroenterol Hepatol 2011;8:512-22.
- Rosen CB, Heimbach JK, Gores GJ. Liver transplantation for cholangiocarcinoma. Transpl Int 2010;23:692-7.
- Dziegiel P, Podhorska-Okolow M, Surowiak P, et al. Influence of exogenous melatonin on doxorubicin-evoked effects in myocardium and in transplantable Morris hepatoma in rats. In Vivo 2003;17:325-8.
- Fan LL, Sun GP, Wei W, et al. Melatonin and doxorubicin synergistically induce cell apoptosis in human hepatoma cell lines. World J Gastroenterol 2010;16:1473-81.
- Deyhimi P, Azmoudeh F. HSP27 and HSP70 expression in squamous cell carcinoma: An immunohistochemical study. Dent Res J (Isfahan) 2012;9:162-6.
- Leja-Szpak A, Jaworek J, Szklarczyk J, et al. Melatonin stimulates HSP27 phosphorylation in human pancreatic carcinoma cells (PANC-1). J Physiol Pharmacol 2007;58 Suppl 3:177-88.